Meeting the building and energy codes with EIFS
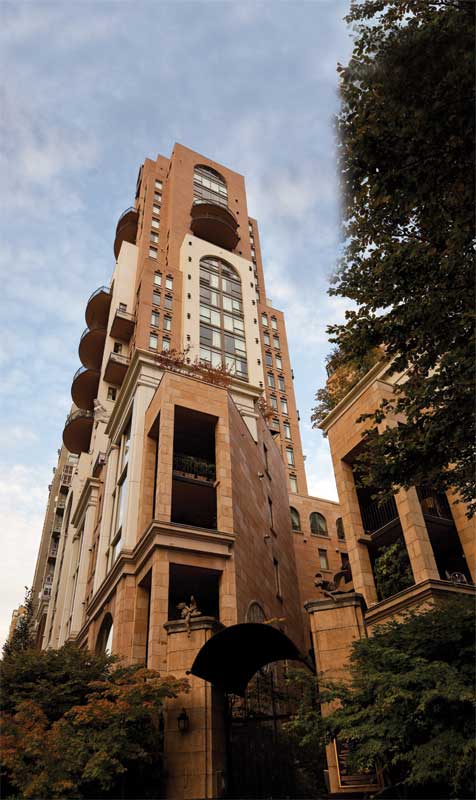
By Amanda Sinnige and Kevin Day, LEED AP
Energy efficiency, green construction, increased insulation, net-zero—all these terms imply the same expectation: to improve the performance of buildings and minimize their impact on the environment by reducing greenhouse gas (GHG) emissions, conserving natural resources, and maximizing the service life of all building systems—all while maintaining comfortable and functional buildings. This is a tall order, but in an age where our impact on the environment can be measured by the legacy left for future generations, design/construction professionals have a responsibility to ensure every building constructed minimizes its ecological footprint. In other words, net-zero buildings are not ‘nice to have’ but an urgent necessity.
To understand how to meet the requirements of energy efficiency codes, it is helpful to review the history of energy code development for buildings in Canada, and to examine the concept of thermal bridging. It is also important to be mindful of the requirements of individual locales, since these vary by the provincial code adoption cycle. Ultimately, all these factors show how exterior insulation and finish systems (EIFS)—the original continuous insulation—can be used to successfully meet the requirements of building and energy codes.
Energy consumption of buildings
Engineering and management firm Morrison Hershfield aptly describes the impact of building energy usage in its Building Envelope Thermal Bridging Guide:
Space conditioning, primarily heating, is one of the largest components of energy use in commercial, institutional, and residential buildings in B.C. Building envelope thermal performance is a critical consideration for reducing space heating loads and will be an increasingly important factor as authorities strive for lower energy consumption in buildings. (For more information, consult the Building Envelope Thermal Bridging Guide – Analysis, Applications, and Insights by Morrison Hershfield Ltd. [2014].)
According to Natural Resources Canada (NRCan), the residential sector accounted for 17 per cent of national energy use in 2013, with the commercial/institutional sector accounting for 10 per cent (Figure 1). (Further reading on these statistics is available at www.nrcan.gc.ca/sites/www.nrcan.gc.ca/files/energy/pdf/trends2013.pdf.) Total secondary energy consumption for all sectors was 8924 PJ. In the same year, space heating in the commercial/institutional sector accounted for 55 per cent of energy consumption, as shown in Figure 2.
Considering these statistics, reducing thermal bridging is more than a good idea—it is critical to reducing the impact buildings have on the natural environment.
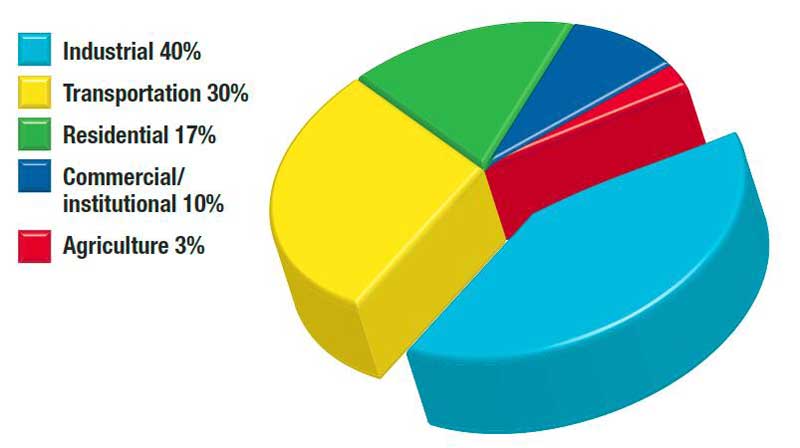
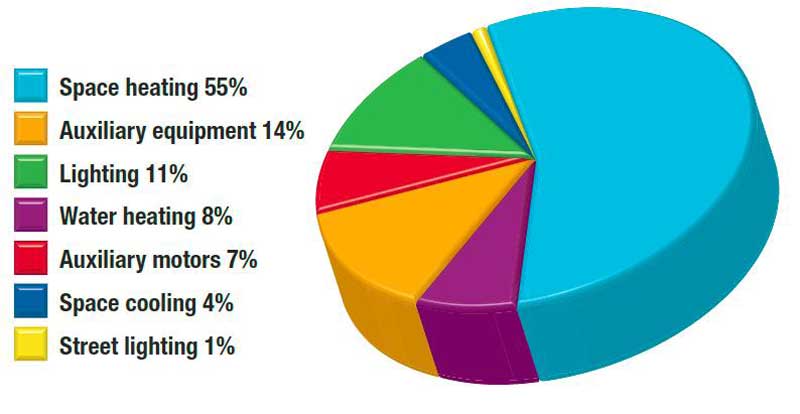