DC: The power to change buildings
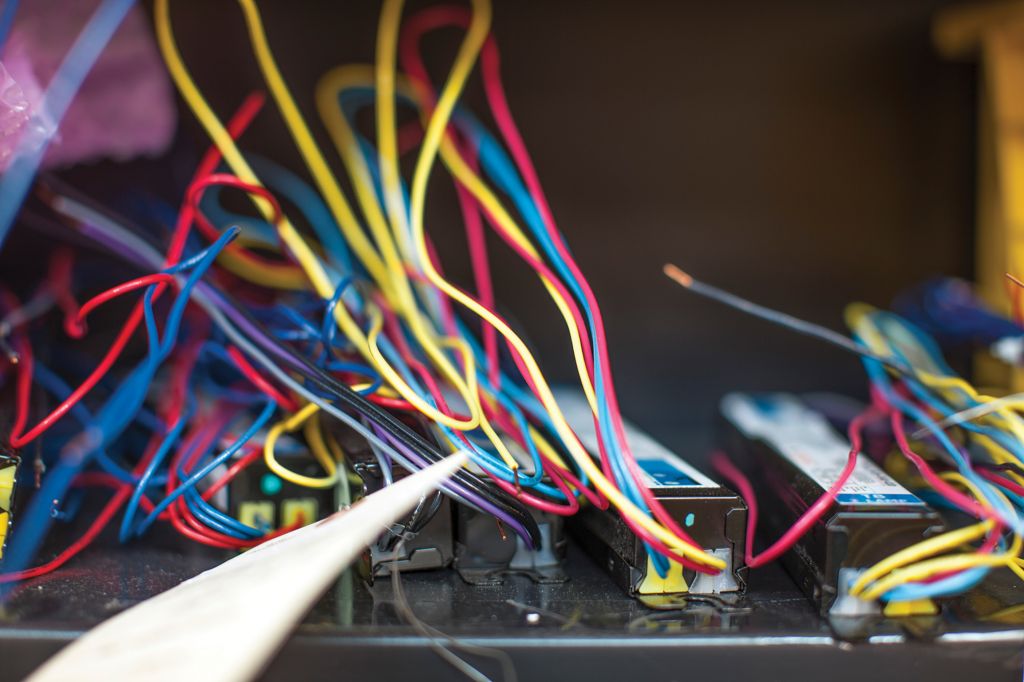
By Brian T. Patterson
Alternating current (AC) power won the ‘Battle of the Currents’ more than a century ago, but is it still the most practical means of power distribution? Certainly, AC power has been a reasonable choice for power distribution in the past, but due to changes in power generation, storage, and use, combined with advanced electronic technology and motivated by groups looking to move away from overdependence on traditional fossil fuel sources to cleaner alternative sources, it is quickly becoming apparent direct current (DC) could be making a comeback.
Indeed, for reasons explored in this article, nature’s original form of electricity has the power to change the built environment for the better. However, what should design/construction professionals know about DC power to ensure its systems can be best incorporated? 1
Keeping current with AC/DC terminology
Alternating current is electrical current that reverses direction at a frequency of either 50 or 60 times per second (Hz). AC is created at relatively low voltages (i.e. 11 to 33kV) by central plant generators, stepped up to higher voltages by transformers, distributed at high voltages (110-kV and above) across large distances, and then gradually stepped down to lower voltages (13 to 16 kV) by transformers for community-level distribution and again to even lower voltages (110 to 480 V) for use in homes and businesses.
In order to connect multiple AC generation sources into the same grid, all sources must be voltage-regulated and synchronized to the exact same frequency and phase as the primary source. This usually involves careful regulation of each generator or by the conversion of the AC power generated to DC, so it can be electronically ‘synchronized,’ and converted back to AC for coupling.
As its name suggests, direct current always travels in the same direction. This type of current can be created by generators, batteries, fuel cells, solar panels, and wind turbines. Multiple sources of DC can be easily coupled by regulation of the DC voltage.
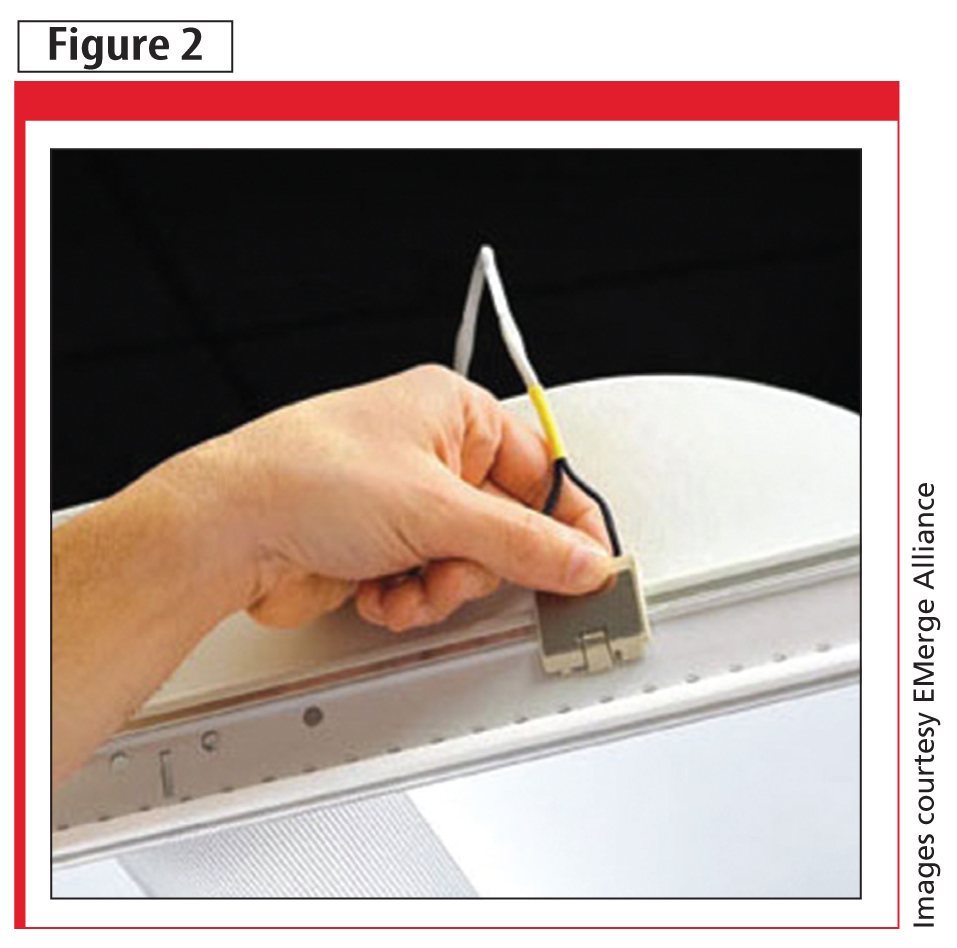
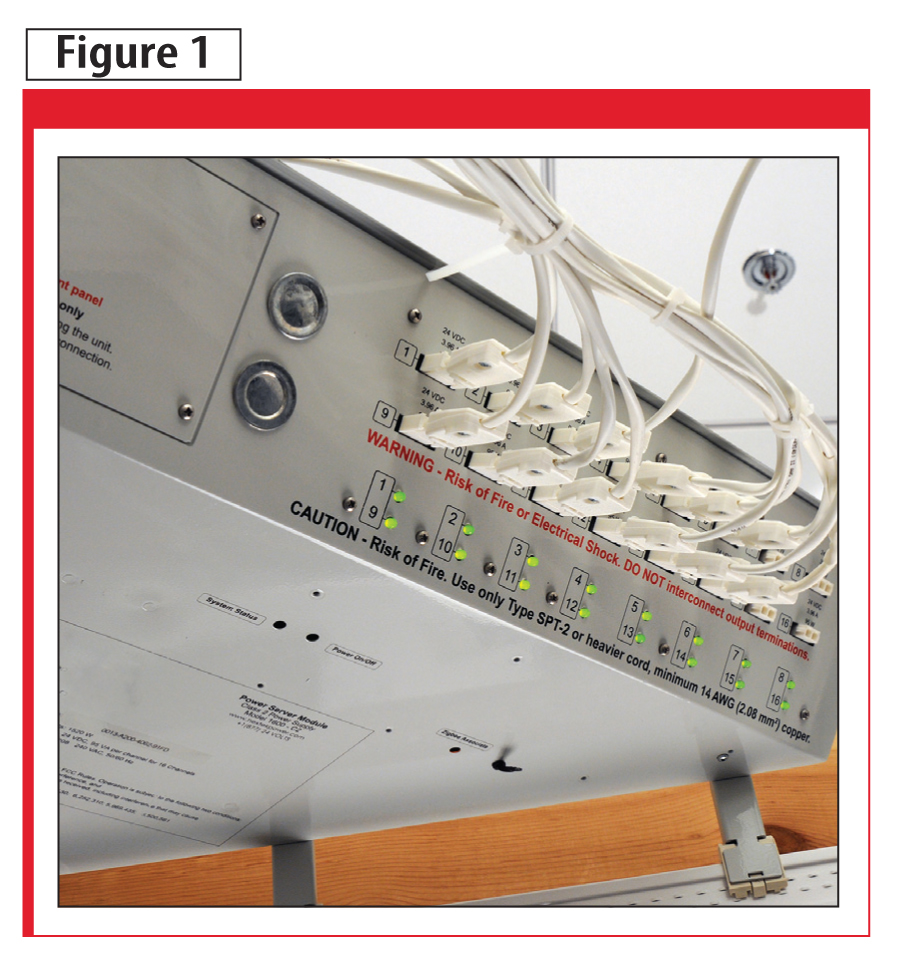
Primary voltages in a DC grid or system are electronically transformed and regulated typically using modern isolated gate bi-polar transistor technology. Using modern electronics, most forms of efficient long-distance transmission of electricity is done using DC power due to the reduced interferences caused by alternating magnetic fields produced with AC.
It is generally known that at identical voltages, DC power has better transmission characteristics. Since most site-based devices that generate alternative power, such as solar panels, wind turbines, fuel cells, and batteries produce either DC or non-frequency controlled AC, the power they generate is easily coupled using simple voltage controls and can be distributed directly to building devices without conversion.
Older electrical (non-electronic) devices were designed to operate in a fixed or non-variable mode on 50 to 60 Hz frequency AC power (typically AC motors) or by acting as simple resistors, such as heaters or incandescent lights. However, almost all modern electronic devices require DC electricity—these include computers, solid-state lighting (i.e. light-emitting diodes [LEDs]), phones, personal electronics, robots, variable frequency drives, and other factory machinery and anything else digital.
Limitations of alternating currents
With today’s technology-driven world, AC power’s limitations are quickly being realized. Currently, more than 70 per cent of the generated AC power needs to be converted into DC power for use in electronic devices and systems. Alternative energy sources, such as solar and wind power, are also native DC-producers, making the conversion process to AC and then back again to DC all the more tedious and ineffective.2
Among the biggest drawbacks to using AC power is the inefficiency and loss of electricity suffered when converting it to direct current. Today’s buildings are powered by traditional AC sources, but are populated with DC-powered devices such as computers, phones, and IT equipment. Even lighting systems, which have historically been AC-driven, are becoming more natively DC-powered as light-emitting diode (LED) fixtures and advanced lighting controls become more prevalent in various commercial spaces.3
Wiring buildings exclusively with AC electric distribution requires every device that uses DC power to include an adaptive means to convert the delivered current to the required one. This process consumes from five to 50 per cent of the power converted.4 Further, every wasted watt of electricity becomes heat, which most often puts a further energy requirement for cooling. By creating building spaces with DC electric distribution, one avoids the need for this conversion, making onsite electrical devices more efficient.
Advantages of DC distribution
Using DC distribution offers advantages to building operators, in addition to helping meet energy reduction goals. It ‘future-proofs’ sites by enabling addition of alternative energy sources through provision of easy application of multisource generating and storage systems. By eliminating the need for power inversion to feed the local building requirements, these distribution systems provide a more efficient way to power commercial spaces and buildings.
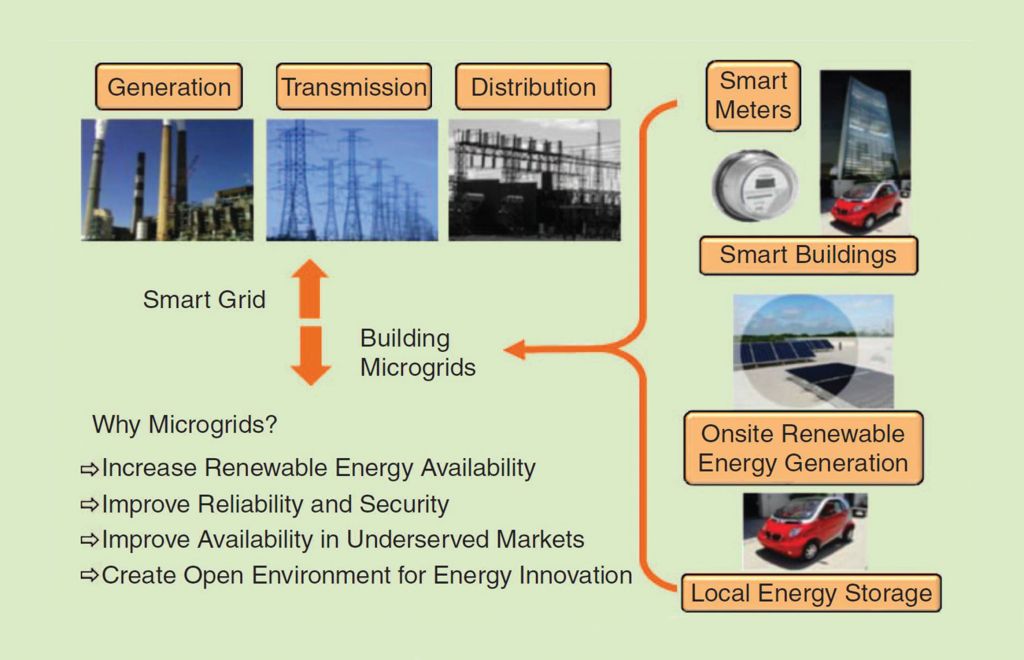
Energy savings
While the amount of savings in electrical energy is somewhat difficult to completely capture, a Yale-published research estimate puts the annual potential reduction at more than eight per cent of the total national electric load, or approximately 400 million kWh annually.5 This does not take into account additional energy savings as a result of less cooling being required in buildings where fewer electronic conversion devices are used; it also does not account for losses associated with conversion of alternate generation from DC to AC.
Renewable energy integration
DC microgrids (discussed later in this article) facilitate simple and more energy-efficient coupling (eliminating the need for e-inverters/converters) of distributed alternate and renewable-energy generators (e.g. solar, wind, fuel cell, etc.) that natively produce DC power. Industry-sponsored research at the Electric Power Research Institute (EPRI) has concluded multiple distributed source generation coupled onto a common DC busbar (bus) can simplify and make more efficient use of site-based alternate energy.6
Energy storage
Local energy storage will be essential to widespread use of non-dispatchable distributed energy-generators. The distributed bus architecture of DC microgrids allows simple and more energy-efficient coupling of natively DC electricity storage such as batteries and ultracapacitators.
Control and monitoring
Most modern controls, sensors, and monitoring systems natively run on DC current and are digitally and/or microprocessor-based. The use of DC microgrids therefore greatly simplifies the electronics involved with electrical and energy system control and monitoring by eliminating the need to convert power to operate.
System reliability
The intrinsically simplified, disaggregated topology of DC microgrid networks ensure a higher level of basic service reliability. In other words, having a system made up of a large number of smaller, self-sufficient ‘sub-grids’ is inherently more resistant to large, catastrophic failure.
By being ‘islandable,’ both the public grid and the individual DC microgrids can be isolated from one another during other dynamic and damaging linear failure events and disturbances. The simplification in subsystem and device-level power conversion/inversions generally improves ‘mean-time-to-failure’ levels in the equipment (i.e. with less electronic stages and components, one can avoid more points of failure).
Without unnecessary power converters, electrical devices are more efficient, portable, and reliable. Individually pluggable and addressable devices can be reconfigured as quickly as occupants’ needs change. The platform is intrinsically safer and more flexible than an equivalent AC system, and thus allows more flexible and user-friendly plug-and-play capability.
Electric vehicle integration
Power grids will increasingly need to accommodate the shift in energy distribution and use caused by the rising number of electric vehicles and their need for plug-in charging (and occasional use as supplemental storage or electricity backup capability). Since these vehicles run on DC electric systems, direct-current microgrids will more easily provide for their charging (including fast charging) and storage connectivity needs—again with fewer wasteful power conversion/inversion steps.
Power of DC microgrids
As shown in Figure 1, a microgrid is an electricity distribution system containing loads and distributed energy resources, such as:
- distributed generators;
- fuel cells;
- renewables;
- energy storage devices; and
- controllable loads.
These components can be operated in a controlled, co-ordinated way while connected to or disconnected from the main power network.
Considered by many experts to represent the ideal building blocks of a far more resilient and efficient ‘smart grid,’ DC microgrids offer the local ability to couple multiple sources of power without complex frequency synchronization, phase correction, and line balancing while better matching native DC power sources with their predominantly native loads, minimizing conversion complexity and efficiency losses. As a result, microgrids behave more robustly in the face of blackouts and natural disasters, terrorist attacks, or human error.
DC power in effective building operation
Specifying a direct-current infrastructure promotes green construction and facility management practices by enabling use of simpler devices that are DC-native. However, from a building operation and maintenance perspective, the benefits of DC power grids go beyond going green and decreasing energy consumption by reducing installation, renovation, and maintenance labour costs and business interruptions.
With a distributed power grid bringing low-voltage DC power throughout a space, several safety and maintenance practices become much easier. When a need to change furniture or traffic patterns in an area arises, a modification in the lighting design often comes with it.
This might typically require significant rewiring or new circuits and re-commissioning of control systems by skilled electricians, including provisions for temporary lighting while the system is being reconfigured. With a DC power distribution system, however, the pluggable busses are already distributed throughout the space—only a physical reconfiguration of fixtures and possible software reprogramming would be required, particularly with today’s wireless control systems.
Additional or upgraded fixtures may be easily added without new wiring, since power would be drawn from the electrified ceiling grid already in place. At safe low voltage, luminaires (like the one in Figure 2) may be relocated without the need of shutting down the entire circuit, a technique called ‘hot-swapping,’ and the fixtures do not require separate high-voltage disconnects. Where the task is simply troubleshooting or repairing a single fixture, safe and speedy maintenance can take place without disruption to normal business operations.
Accelerating net-zero attainment
The possibilities of the application of DC power are limitless. While most applications today already suggest the integrated use of direct-current microgrids in commercial buildings, the future of DC power lies in the creation and development of zero-net-energy buildings (ZEBs) that ‘cleanly’ generate enough energy onsite to equal the energy they use, creating a balance at the building level.
When it comes to energy—and especially electrical energy and the marvel of our existing century-old AC electrical energy system— many are tempted to disown the challenge of creating a better future as represented by this more balanced vision of the role DC can play. However, what DC proponents are envisioning is no more dramatic, demanding, or risky an undertaking than that associated with the recent transformations of our telephony, information, and computing systems during the creation of the Internet. In some ways it should be a far less ominous transformational job, as the lessons learned from crafting the World Wide Web are still fresh in minds. The reward: an electricity network that can enhance business and personal economic growth and ecological well-being in a way that rivals the positive effects of the Internet.
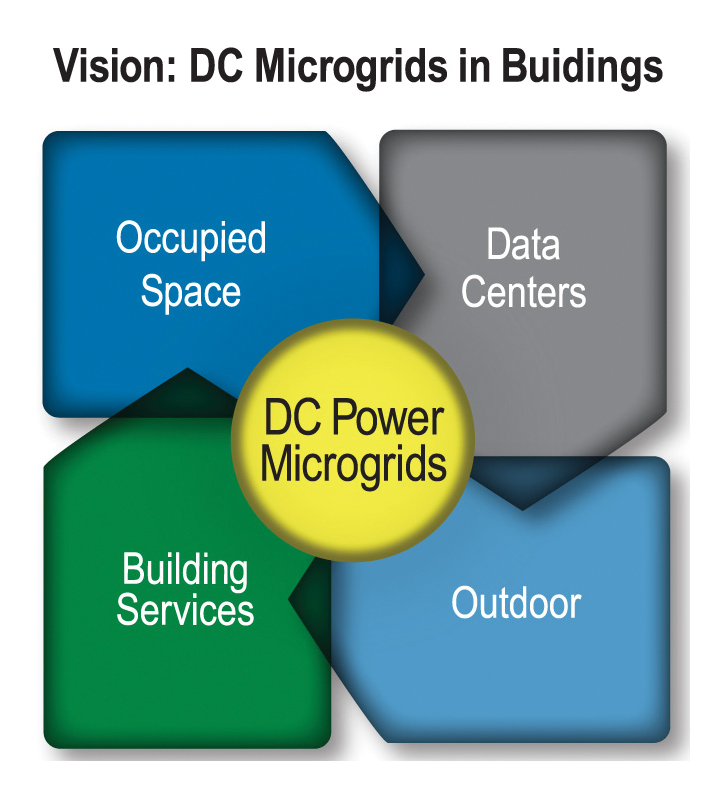
While the goal is to develop entire campuses and business complexes that are reliable, sustainable, and run entirely off of DC power, there are still issues that need to be addressed in the immediate future. One of the biggest challenges is we are not starting from scratch.
We cannot just concern ourselves with new buildings—of all the commercial buildings that will exist in 2030, 85 per cent are already built. Ultimately, as technology and ideas advance, so should our focus to find ways to improve the way we power existing buildings, as well as future ones. By allowing easily interconnected scalable DC microgrid networks, some as small as a single room or floor, to be deployed opportunistically, as time, money, or need arises, DC distribution systems can provided the perfect solution to this otherwise daunting task.
Planning for the future
The EMerge Alliance’s goal is to have at least all new construction and an equal number of major renovations at net-zero running on hybrid DC microgrids by 2030. The beauty of the approach to implementing a DC infrastructure is it need not be an ‘all-or-nothing’ proposition.
Like computer networks, DC microgrids can be implemented at anywhere from the room level to an entire campus. That is, the DC distribution is made up of a number of sub-layers that, when interconnected, form the entire microgrid. This allows a practitioner to implement the technology in stages, perhaps opportunistically based on resources and needs, over time. The Internet took well over 40 years to evolve to what it is today, and some people would argue it still is not complete.
Another good comparison would be our telephony system. Despite the heavy use of cell phones and voice over Internet Protocol (VoIP [e.g. Skype]), most Canadian homes and offices still have a traditionally wired system, at least in part. Looking to newer installations (or those in places where existing infrastructure is unavailable), pure cellular and IP-based systems prevail. Again, a hybrid use of traditional and new technology will be in use for some time. The alliance sees the power domain going down the same path, perhaps at a brisker pace since all the technology to do so is already available, which is something the Internet and VoIP systems had to develop in parallel and over a longer period.
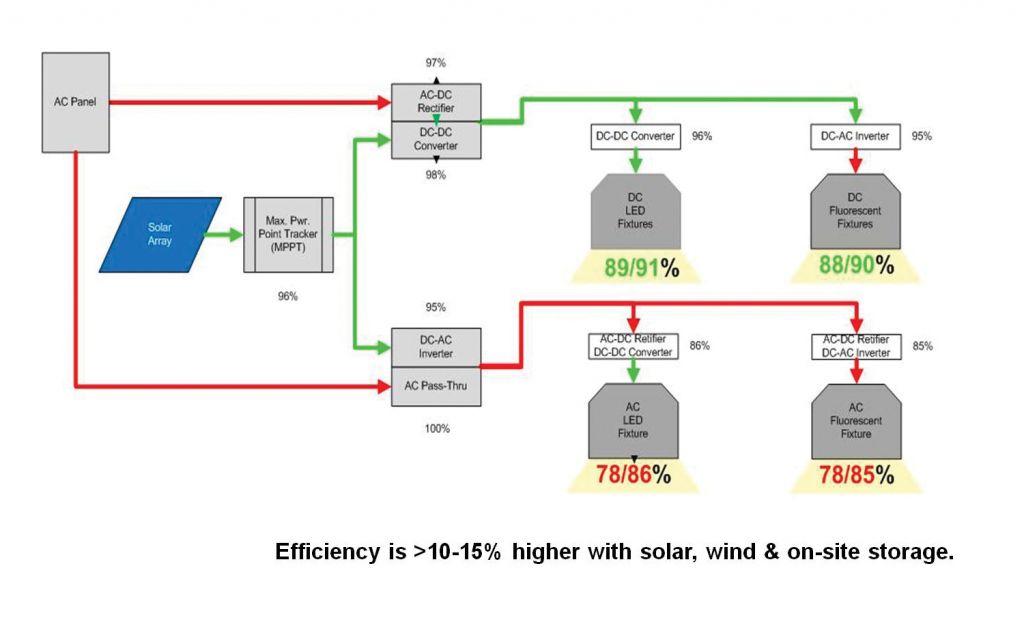
Hybrid power distribution will be the majority of implementations, even by 2030, simply because of the significant installed base. The motivation for building owners, architects, designers, specifiers, and managers will be to not over-invest in older technology that will meet with premature obsolescence due to the newer technology offering increasingly higher performance and decreasing total ownership cost over time. While the velocity of this technology transformation has yet to be determined, the directional trend is as clear as its advantages.
The Alliance is working to create standards for DC power throughout buildings. But notwithstanding the temporary absence of those standards, several demonstrations have substantiated the assertion that all the technology necessary is already available. The remaining issue is the marketing of suitable DC-connected loads (devices that accept a direct DC input that eliminates or by-passes the traditional AC input).
Due to the lack of standards, companies have been reluctant to provide this feature without knowing what DC supply voltages and interconnection means are available. The only de facto standards thus far have been provided by the automotive industry (mostly 12Vdct) and at the device level (mostly 5Vdc) by the personal electronics industry. These voltages are not suitable for general distribution of power in commercial buildings due to their excessive low voltage wire loss over longer distances. Therefore, while many companies are moving ( or would like) to market devices directly powered by DC, the selection is still limited.
The EMerge Alliance has taken the lead in correcting this situation by creating higher distribution voltages. Once appropriate voltages and interconnects are defined for all commercial applications, the original equipment manufacturer (OEM) market will develop completely. In the meantime, the alliance maintains an active registry and encourages companies that do make suitable equipment to list their products to existing Alliance standards there. This will be an ongoing process of collecting and providing such information and education over the coming years.
Notes
1 This author has adapted the article from his presentation at the 2013 CSC National Conference in Calgary. (back to top)
2 Although wind seems like it could be ideal for AC power generation, maintaining the 60-hz frequency of its output is a significant issue. The wind is inherently variable, so unmodified, the frequency of the naturally produced AC will also be variable—thus without further conversion, it has little practical use. In today’s AC power systems, frequency must be adjusted and phase-synchronized to exactly match the final distribution system’s frequency. The most practical and economic way to do this is to convert the highly variable frequency AC power originally produced by the wind turbine to DC so it can be electronically manipulated/corrected. The question is, of course, why take the electronic losses to convert the DC back to AC when it can be used directly as DC? For more information, see the U.S. Department of Energy’s (DOE’s) 2010 Litos Strategic Communication, “The Smart Grid: An Introduction.” Visit www.oe.energy.gov/DocumentsandMedia/DOE_SG_Book_Single_Pages.pdf. (back to top)
3 For more on LEDs, click here. (back to top)
4 Small devices generally have the worst conversion losses since a higher fraction of the total power consumed is by the conversion electronics; conversely, larger devices tend to use a smaller fraction of the total for conversion electronics. Generally speaking, manufacturers of larger power-consuming devices spend more money for better power-conversion electronics. The reality is the total power consumed is increasingly made up of small, individual, powered devices—computers, printers, and personal electronics—instead of big factory pumps and motors. (back to top)
5 See the report, “DC Microgrids: Benefits and Barriers,” by Paul Savage, Robert R. Nordhaus, and Sean P. Jamieson in the 2010 book, From Silos to Systems: Issues in Clean Energy and Climate Change. (back to top)
6 A busbar is a strip or bar of copper, brass, or aluminum that conducts electricity within an electrical apparatus. It is used to distribute power, and generally allows connection of either sources or loads along its length. For more on the report, visit mydocs.epri.com/docs/CorporateDocuments/WhitePapers/EPRI_DCpower_June2006.pdf. (back to top)
Brian T. Patterson is general manager of Armstrong World Industries, and chairs the EMerge Alliance—a non-profit corporation established to create application standards for direct-current (DC) power distribution systems use in buildings. The group also promotes the development of an environment to support the adoption of those standards, including design/construction professionals, building owners, policy/regulatory/compliance officials, and original equipment manufacturers and system integrators. He can be contacted via email at btpatterson@armstrong.com.
To read about EMerge Alliance Moving DC Power Forward, click here.