Wind design for modular vegetated roofing systems
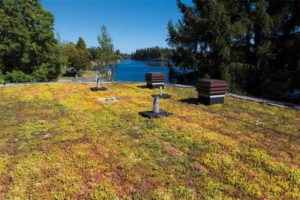
By Karen Liu, PhD
Vegetated or green roofs offer multiple benefits to urban areas. They help manage stormwater, reduce energy demand, mitigate urban heat island, improve air quality, and enhance biodiversity.
As a result, many municipalities are offering various forms of incentive programs to encourage green roof adoption. In 2009, the City of Toronto adopted the Green Roof Bylaw and became the first city in North America to require green roofs on most new buildings covering 20 to 60 per cent of the available roof space based on the building’s size. Additionally, it also offers $100/m2 to encourage green roof installation through its Eco-Roof Incentive Program. Since then, more than 600 green roofs covering 465,000 m2 (5 million sf) collectively have been constructed in Toronto, and it has become one of the top North American municipalities with most green roof constructed.
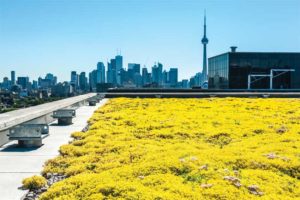
Photo © Greg Van Riel Photography
Many green roofs in Toronto are located on high-rise buildings and lakefront properties that experience high wind (Figure 1). They must be secured against wind forces to meet performance requirements and ensure public safety. The Toronto Green Roof Construction Standard governs the design and construction of green roofs and sets out the minimum requirements to meet the city’s objectives. On the issue of wind, the standard requires an engineer-stamped report providing design wind pressures for green roofs and outlining how they are addressed. However, technical information on wind resistance of green roof was lacking.
To address this gap, the National Research Council Canada (NRC), in collaboration with the members of the Canadian green roof industry, conducted a three-year research project to study the wind performance of green roofs. The research findings led to the development of a new national standard—the Canadian Standards Association (CSA) A123.24-15, Standard Test Method for Wind Resistance of Modular Vegetated Roof Assembly, which evaluates the wind resistance of the vegetated roof assembly and enables designers to select the appropriate system for their projects.
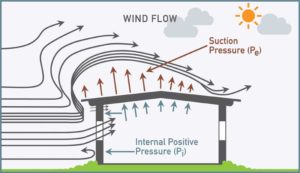
Images courtesy Next Level Stormwater Management
Wind effects on vegetated roofing assemblies
When wind hits the wall of a building, the air moves upward and increases its speed and creates positive pressure on the wall. The air continues to travel up and over and creates a negative suction on the roof (Figure 2). The distribution of the wind forces is non-uniform across the roof, with considerably higher localized forces in the corner and edge regions than the field of the roof. While the magnitudes of these pressures vary with the wind speed, the spatial distribution remains.
The negative pressure or wind-induced suction affects both the roofing system and the vegetated system, but in different ways. A roof membrane is impermeable and attached to the roof structure, so the wind uplift pressure creates suction that lifts it like a sail. In comparison, a vegetated system is unattached to the roof structure and is permeable, akin to a sail with tiny holes. Wind can move through the green roof, which creates pressure equalization, thus reducing the net overall uplift.
Intensive green roofs consist of large plantings such as trees and bushes thriving in deep, growing media with saturated weight typically ranging from 200 kg/m2 (41 psf) to more than 1000 kg/m2 (205 psf). Wind uplift is usually not a major concern due to the heavy weight. Nevertheless, for exposed rooftops on high-rise buildings where high winds are expected, it is recommended to anchor the trees, or the root balls, to the structural elements to prevent blowing off.
Extensive green roofs contain small vegetation, such as sedums, grasses, and perennials growing in shallow growing media with design loads typically in the range of 50 to 200 kg/m2 (10 to 41 psf). These lightweight systems are more affected by wind (Figure 3). Additionally, exposed growing media are prone to scouring and displacement, particularly in the corner and edge regions of the roof where localized wind forces are higher. As typical green roof growing media are mineral-based and granular in nature, they are more likely to be displaced than cohesive growing media with higher clay contents. Erosion control nettings, tackifiers, and hydromulches can be used to reduce wind erosion, particularly during the establishment stage when the vegetation has not yet rooted firmly into the growing medium.