Role of HVLS fans in disinfecting indoor air
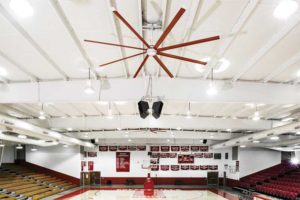
By Alex Risen
Using an overhead high-volume, low-speed (HVLS) fan’s bi-directional airflow and elevated air speed is a powerful way to keep occupants comfortable in applications ranging from non-conditioned industrial spaces to commercial buildings. Immediately underneath an HVLS fan, airflow is pushed downward. Outside its diameter, the airflow transitions to horizontal air movement, providing comfortable airflow over a large area, according to thermal comfort calculations as per the American Society of Heating, Refrigerating and Air-conditioning Engineers (ASHRAE) 55, Thermal Environmental Conditions for Human Occupancy.
Directional fan and airflow
Directional fans create a one-way airflow pattern that moves air to the area in front of the fan. Directional fans are most often used to cool occupants in congested areas where overhead fans cannot be safely mounted. Additionally, premium directional fans feature variable speed control, allowing users to adjust airflow to provide optimal comfort.
Improving ventilation with fans
Fresh air is not typically distributed uniformly throughout a space. For example, one of the most common air distribution configurations for HVAC systems is an overhead supply with overhead return. In common heating applications, this ceiling configuration can cause significant stratification of heated supply air and room air layers, preventing fresh air from circulating to occupant level. To compensate, ASHRAE 62.1, Ventilation for Acceptable Indoor Air Quality, requires the increase of ventilation rates by 20 per cent to deliver the necessary amount of fresh air to the room’s occupants. However, in a room destratified by an HVLS overhead fan, the air layers are well mixed so the supplied fresh air can reach the occupant level.
In naturally ventilated spaces, fresh air is passively distributed throughout the space, so localized areas may have stagnant pockets, resulting in poor air quality and buildup of pollutants. Fans disperse these pockets and increase the circulation of fresh air, evenly distributing it throughout the space and improving indoor air quality (IAQ).
Impact of increased air movement on ventilation
Using a computational fluid dynamics (CFD) analysis of an industrial space shows the measurable benefits offered by a large diameter, HVLS overhead fan. In one instance, a 7-m (24-ft) model pushes airflow directly downward in an area of roughly 46 m2 (500 sf). Horizontally, the fan is able to push airflow over a larger area (depending on mounting height, floor-level obstructions, fan model, etc.).
Operating fans during flu seasons
Many variables are at play within each facility and application. Without data and intensive study for each case, it is inconclusive whether elevated air speed has a significant health impact on a facility. Following best practices, as outlined by local public health units and the World Health Organization (WHO), in addition to adhering to building design standards and practices set forth by ASHRAE, ensures proper design and implementation of systems.
Ventilation can reduce the concentration of airborne pathogens through dilution. Increased ventilation rates (outdoor air intake) can provide a higher dilution capability and, potentially, reduce the risk of infection. As such, the following are accepted recommendations to consider:
- all facilities should meet or exceed the latest practice standards, including but not limited to ASHRAE 62.1 for ventilation and ASHRAE 55 for thermal comfort conditions;
- in naturally ventilated spaces, it is best to maximize the intake of dilution ventilation (outdoor air) whenever possible and distribute to all building occupants;
- practice good respiratory hygiene, such as occupants should cough or sneeze into an elbow or tissue and practice regular handwashing; and
- clean and disinfect frequently touched objects and surfaces such as workstations, keyboards, telephones, handrails, and doorknobs.
In addition to using fans on their own, applying modified design guidelines, similar to those used for common destratification applications to increase the rate of air circulation between the upper and lower air zones, can improve the performance of upper-room ultraviolet germicidal irradiation (UVGI) systems by up to 77 per cent.
Improving upper-room UVGI system performance with fans
The use of UVGI technologies in the HVAC industry is well established and understood. The technology has been paired with HVAC equipment use since the early 1940s and the benefits they provide reduce occupant exposure to contagions by disinfecting indoor air near specially designed UVGI devices. These devices resemble standard fluorescent tubes or lighting luminaires and can be ceiling or wall-mounted, portable, installed in ducts, or packaged in the equipment itself.
One of the most common applications is upper-room UVGI. Here, the fixtures are installed above the occupied zone and oriented horizontally such that their radiation output (in the form of UV-C waves) is concentrated to create a disinfection zone above the occupant breathing zone (below), limiting the occupants’ direct exposure to elevated radiation levels. The elevated radiation levels in the disinfection zone damage the DNA/RNA of micro-organisms including that of bacteria, fungi, and viruses. With damaged genetic material, the often contagious micro-organisms are inactivated and unable to replicate in the indoor environment or inside of any host. Viruses are the most susceptible micro-organisms to UV-C irradiation, followed by bacteria and then fungi. Upper-room UVGI is the oldest application, the most easily retrofittable one, and is approved for improved control of highly contagious airborne diseases, such as tuberculosis by the U.S. Centers for Disease Control and Prevention (CDC) and the National Institute for Occupational Safety and Health (NIOSH).