Green roofs, cold winters, and dealing with drought
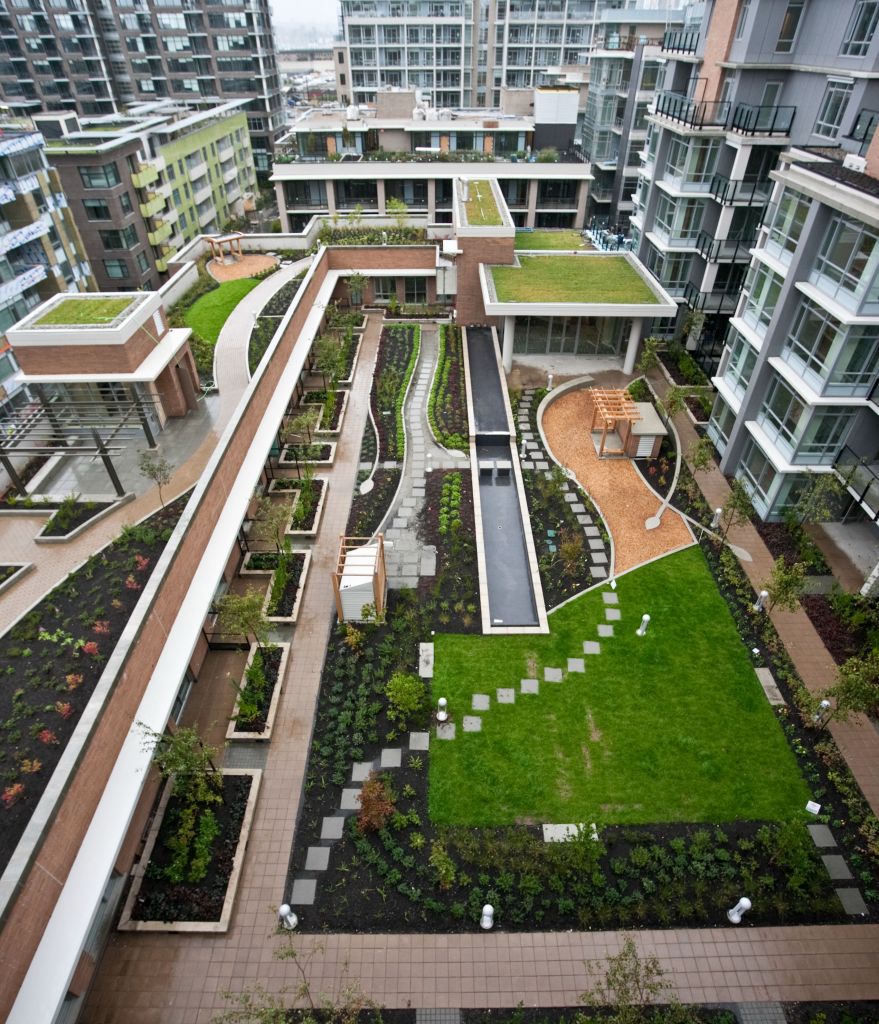
By Shazia Husain, PhD
As its name suggests, a vegetated or green roof includes shallow-rooted plant material that can withstand extreme climatic conditions atop buildings. These assemblies offer numerous potential benefits, from absorbing rainwater runoff and providing insulation, to mitigating the urban heat island effect and providing greenspace.
Intensive vegetated roof systems are thicker and can support a wider variety of plants, but are heavier and need more maintenance than extensive assemblies, which are covered in a light layer of vegetation. Either way, it is important to ensure the right plants are being used for the project. (Along with the usual roofing concerns, such as waterproofing and structural capabilities.)
Following conventional wisdom, green roofing consultants often suggest selecting the plant material native to the project’s locale. This helps avoid unnecessary plant death by way of establishment and/or if the flora is being transported from far away. Native plant species have also already been adjusted to that particular location’s climatic conditions, making it easier for them to get established quickly.
The plants traditionally chosen for vegetated roofs tend to be hardier species that can be grown almost anywhere; they do not require a specific combination of soil material, with some even thriving out of stones. They also need very little water.
While design/construction professionals need not be botanists or horticulturalists, having a background understanding about the subject can be invaluable when working with others to ensure the ideal green roof is being specified. The information shared about the mechanisms of drought and cold adaptations of plants in this article will help engineers and architects designing a green roof understand what type of plants are suitable that are cold-, heat- and drought-tolerant.
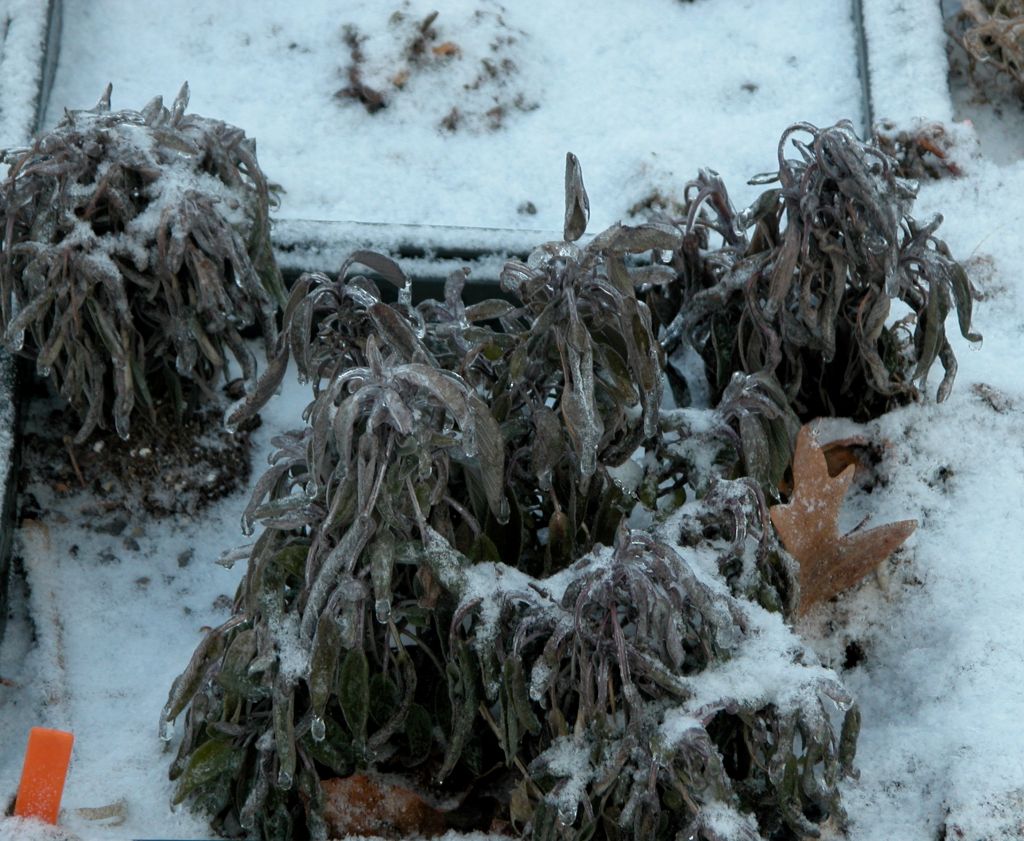
The critical knowledge of rain capture and storage plays an important role on plant survivability on a non-irrigated roof as well. This author has been asked frequently by architects about how long the plants on a vegetated roof live. Many times, manufacturers have to provide a survivability certificate on the type of plants being used on a particular roof and their watering requirements, with information if they are drought-tolerant and can survive in heavy rainfall. To that end, this article discusses aspects of selecting plants with the ability to survive in Canada’s adverse conditions. It also explores how flora can adapt to such situations, with special reference to drought-tolerance and over-wintering effects.1
Plant selection, survivability, and environmental issues
For decades, green roofs have been planted with sedums (e.g. Sedum album, S. acre, S. kamtschaticum, S. rupestre, and S. spurium), but there are few publications on the flora’s basic physiology and water use efficiency. Most sedums are Crassulacean Acid Metabolism (CAM) plants, which means they survive through a photosynthesis method that has carbon dioxide (CO2) fixed by an enzyme (i.e. phosphoenolpyruvate carboxylase [PEPC]) during the night to form malic acid that is stored in the plant cell vacuoles, keeping the stomata closed the next day.2 In other words, CAM plants are characterized by their water use efficiency, making them ideal for rooftop environments where they are unlikely to be frequently tended to by a landscaping team.
It has long been established sedums do not like excessive water, marking them as self-sufficient, drought-tolerant plant species.3 On a sloped roof, irrigation requirements are critical because the system dries out faster than on a flat surface. Transferring mature and well-established plants on a roof is more beneficial for survivability than planting young seedlings.
Vegetative roofing providers are mainly interested in how to successfully grow plants to ensure a smooth transition from the field to the roof. Lack of research regarding planting options and managing green roof survivability in Canadian climatic conditions means this is a topic not yet widely explored. However, over the last decade, various North American universities are undertaking work to study the suitability of plant species using both native and non-native species for Canada’s colder environment.
When it comes to environmental stresses, plants are immobile and therefore cannot avoid harsh conditions the way animals or people can—in short, there is nowhere for them to run or hide. However, many species have developed altering mechanisms for survival in the face of drought, salinity, high and low temperatures, and frost.
Physiological aspects of drought adaptations
Generally, drought is defined as an occurrence of water deficit in the soil or atmosphere. Plant response to drought stress can be classified into three categories:
- drought escape (avoidance);
- dehydration avoidance (postponement); and
- dehydration tolerance.
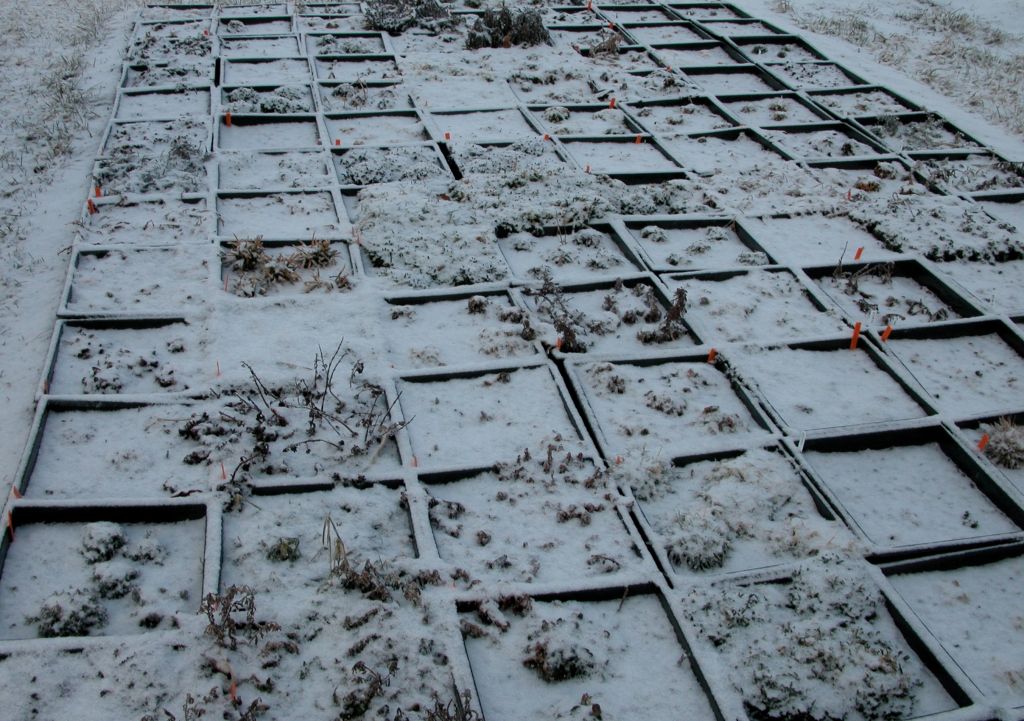
Drought escape
Plants that ‘escape’ drought successfully reproduce before the onset of severe stress. These species (e.g. Sedum reflexum, S. album, S. sexangulare, and S. acre) show a high degree of development flexibility before physiological water deficits occur to complete their lifecycle.4 Advantages and disadvantages of plants with short growth cycle under conditions of water stress and under normal conditions have been reviewed.5
Almost all plant species complete their reproductive lifecycle quickly, producing early flowers, and seeds. To avoid excess energy used in the growth of the plants, the leaf area/leaf area index is reduced, organic reserves are accumulated in different parts of the plants (e.g. stem and roots), which are then remobilized at the time of their reproductive cycle. However, disadvantages of plants with short growth cycles include potentially low seed production and a short period of flowering time. Further, to ensure the plants survive the harsh climatic conditions, they sometimes use their reserve organic material quickly.
Dehydration avoidance
Dehydration avoidance can be defined as where a genotype has higher relative water content than another genotype when both are subjected to the same stress.6 The phenomenon of dehydration avoidance is commonly found in both annuals and perennials involving a number of adaptive traits such as minimizing water loss and maximizing water uptake.7 Many processes result in greater dehydration avoidance such as:
- lower stomatal conductance (i.e. stomata are pores in the plants where gas exchange occurs);
- less leaf area;
- root system deeper in the soil to exploit water availability;
- higher roots/shoots; and
- greater osmotic adjustment.8
The closing of stomata helps reduce water loss and light absorbance through rolled leaves, a dense layer of trichome (increasing reflectance or sloped leaf angles); it also reduces canopy area by shedding older leaves and reduced growth.9 Increasing and adjusting the allocation pattern in the root maximizes the water uptake.10 Also, the shedding of older leaves can be taken as a contribution to water saving by the plant, helping to cleverly distribute nutrients being stored in older leaves to stem and younger leaves.11 Having such qualities means a plant requires less maintenance, and eliminates need for an irrigation system, as the flora can rely on rain for water requirements.
Dehydration tolerance
Dehydration tolerance is a plant’s ability to maintain function as relative water content decreases; it can also be defined as the critical relative water at which plant function stops or starts (e.g. leaf death).12 When the tissue is not protected by any of the avoidance mechanisms, cell loses turgor and dehydrates, resulting in various cellular physiochemical injuries.13 Complete loss of free water will result in desiccation or dehydration. Plants sometimes go into ‘partial dormancy,’ to survive dry seasons.14
Physiological aspects of cold adaptations
Cold stresses include severely low temperatures, strong winds causing desiccation, ice, and pathogens. Some types of plants adapt to these situations and become tolerant; others remain sensitive and eventually die after a certain exposure period. The most common adaptive feature of cold tolerance or overwintering is cold acclimatization with which plants are able to tolerate freezing injury.15 Cold acclimatization involves a number of molecular changes associated with taking care of the membrane functions at low temperatures.16
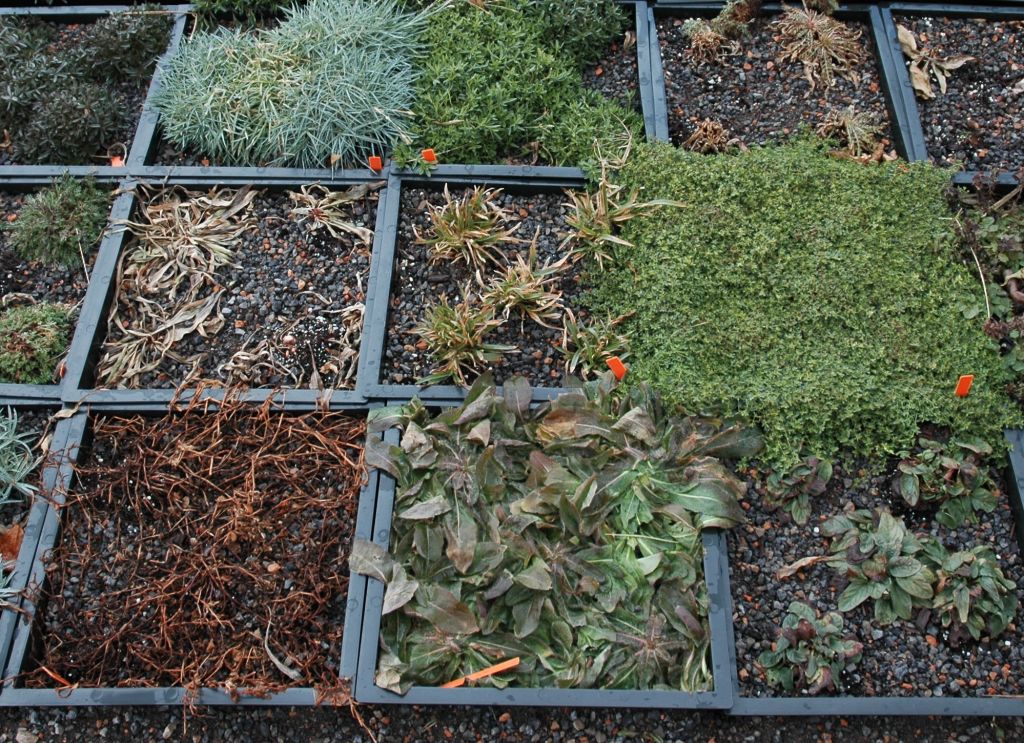
Generally, cold adaptation is defined as plants that survive temperatures as low as −40 C (−40 F) during winter. The freezing of the liquid inside the plants is a serious threat as the freezing of water present in the soil. It causes imbalance in the mechanisms involved in transporting water/nutrients to different parts of the plants. Plants’ response to cold stress can be classified into three categories:
- freeze avoidance;
- freeze tolerance; and
- desiccation injury.
Freeze avoidance
Most of the plants naturally occurring in Canada’s cold climate are acclimatized to begin with in avoiding freezing injury by reducing the chances of ice formation. The problem is more severe within the smaller plant species where they can only super-cool to about −4 to −6 C (25 to 21 F), which is adequate only to avoid few early frosts. In most of the higher plants (i.e. tree species used on intensive roofs, such as Acacia dealbata, Cercis siliquastrum, and Ptelea trifoliata), xylem tissues are damaged when ice formation occurs inside. As this is what facilitates a plant’s water transportation, it leads to a bursting of the plant’s cells and, eventually, its death.
This is important information for architects and specifiers. When winter is about to set in, it becomes critical to ensure the green roof will not be saturated with water—any excess water should be drained out as it can damage the plants when ice is formed. If this is not taken into account, when the snow melts, plants may not revive completely, leaving patches of dead plants on the otherwise-green roof.
Freeze tolerance
Freeze-tolerant plants undergo several changes before cold weather sets in. These changes normally occur inside the tissues where ice can be formed. To keep the plant’s metabolic activities active, soluble sugars are produced as a factor of cold-induced accumulation, contributing to freeze-induced desiccation tolerance by helping stabilize membranes.17 When the plants are acclimatized to cold temperatures, it is absolutely necessary to keep them in a cold-hardened state and that they have enough reserves in them to revive their normal growth features in spring.
Plants need energy for their growth and development to complete their lifecycle in a timely manner. The remaining green, flowers, and seeds in winter plants survive by way of producing sugars to be used as reserved when under the snow cover to keep them growing (though the rate may be slow), returning back to their normal growing activities in spring. Organic reserves (e.g. carbon and nitrogen) play an important role in winter stress tolerance and re-growth in spring.18
When exposed to subfreezing temperatures via rain, decreasing snow, or occurrence of freeze-thaw cycles, the resulting ice formation can mean irreversible injury to plants. Therefore, adequate snow cover (and its insulating properties) is especially important for herbaceous perennials. Due to all these reasons, plants also go into an anaerobic condition, causing depletion in cell energy.19
In respiration mechanism, carbon is released as carbon dioxide gas by the process of respiration. Respiration takes place in plants involving the breakdown of carbon-based organic molecules into carbon dioxide gas. During subfreezing temperature, this process is affected badly because the plant has been in a state of anaerobic shift using excess amounts of carbon and nitrogen reserves.20
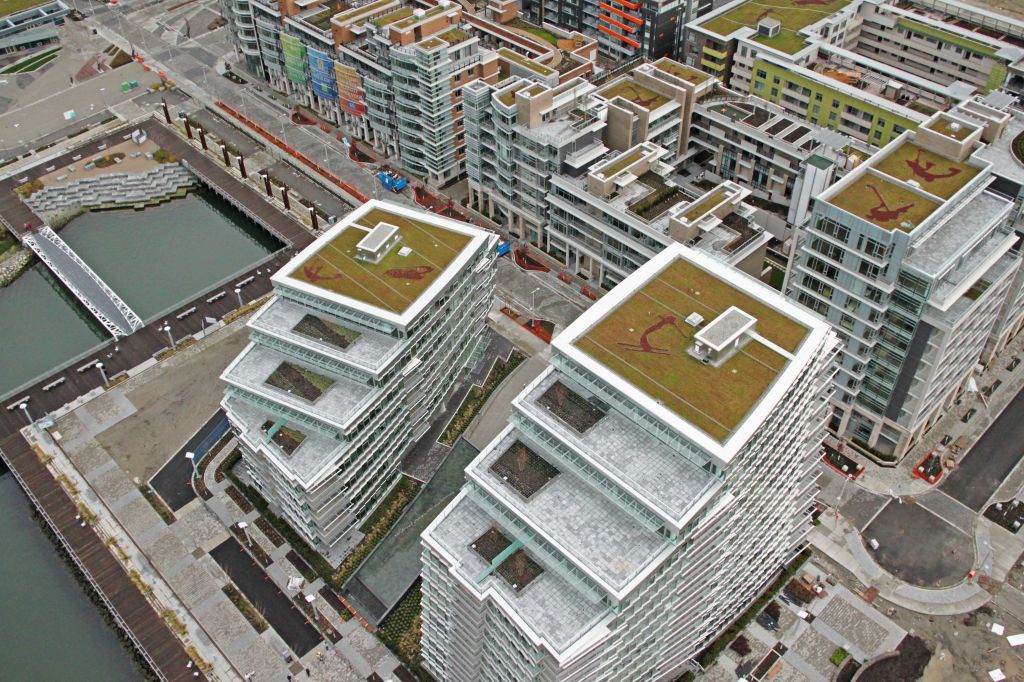
This information can be useful to design/construction professionals in regard to pre-winter maintenance. The specifiers can have information available explaining how to protect the plants from getting subjected to freeze-thaw cycles—essentially instructions not to water, remove any excess water, and avoid ponding.
Desiccation injury
When the water is lost from plants rapidly into the atmosphere and is not replaced by the water present in the soil to the roots, desiccation injury occurs. In winter, humidity is normally low, whereas leaf and air temperatures are high—therefore, the moisture loss is greater and the injury is massive.
Pathogen interaction to cold adaptation
Pathogens attack plants subject to over wintering at sub-zero temperatures. Longer duration of snow cover provides a humid atmosphere below zero temperatures, which is a good breeding ground for pathogens.21
Conclusion
People working for the green roof industry have a huge task ahead of them to understand the effects of cold and drought on the mechanisms of growth and development of sedum plants.
Some of the information provided in this article may not be directly related or relevant to architects and specifiers, but a general knowledge about plants and their mechanisms helps in understanding why a green roof may not be able to establish itself in adverse and harsher climatic conditions.
This information may ease some of the concerns architects and engineers have when approving a green roof on a commercial or a residential building in reference to the types of plants selected and their survivability factors. Research scientists can also help in unravelling the concepts through investigating and resolving factors behind these environmental issues.
Although sedums are considered the most tolerant of plants, like any other living species they are subject to limitations after a certain threshold. It is essential now to investigate the undiscovered fundamental principles of drought and cold tolerance of sedums plants. The information is important for the growers mainly to have a clear idea when planting sedums in terms of survivability culminating into better production enhancing yield, which will be economically beneficial in the end.
Notes
1 This author is grateful to all the authors whose ideas and work helped in putting together this article, especially Professor Rob Berghage, who allowed photos at the Centre for Green Roof Research (Pennsylvania State University), and Dr. S. Shahid Husain (Rtd.) of DG PCSIR labs, who provided critical comments in improving this article. (back to top)
2 For more, see J. Smith and K. Winter K’s 1996 paper, “Taxonomic Distribution of Crassulacean Acid Metabolism,” along with their “An introduction to Crassulacean Acid Metabolism” article in volume 114 of Crassulacean Acid Metabolism: Biochemistry, Ecophysiology, and Evolution. (back to top)
3 See Monterusso et al’s 2005 article, “Establishment and Persistence of Sedum spp. and Native Taxa for Green Roof Applications” in HortScience (vol. 40). See also A.K. Durhman and D.B. Rowe’s “Effect of watering regimen on chlorophyll fluorescence and growth of selected green roof plant taxa” in the subsequent volume. (back to top)
4 See M.M. Chaves et al’s 2003 article, “Understanding Plant Responses to Drought: From Genes to the Whole Plant,” in Functional Plant Biology (vol. 30). (back to top)
5 See A. Blum’s Plant Breeding for Stress Environment (CRC Press, 1988). See also W.R. Jordan et al’s 1983 article, “Strategies for Crop Improvement for Drought-prone Regions,” in Agricultural Water Management (vol. 7) and L.P. Reitz’ 1974 article, “Breeding for More Efficient Water Use: Is it Real or a Mirage?” in Agricultural Meteorology (vol. 14). (back to top)
6 See E.A. Hall’s 1993 article, “Is Dehydration Tolerance Relevant to Genotypic Differences in Leaf Senescence and Crop Adaptation to Dry Environments? Plant Responses to Cellular Dehydration during Environmental Stress” in the American Society of Plant Physiologists’ Plant Physiology. Visit www.plantphysiol.org. (back to top)
7 See note 4. (back to top)
8 See note 6. (back to top)
9 See J.R. Ehleringer and T.A. Cooper TA’s 1992 article, “On the Role of Orientation in Reducing Photoinhibitory Damage on Photosynthetic-Twig Desert Shrub,” in Plant, Cell, and Environment (vol. 15). (back to top)
10 See R.B. Jackson et al’s 2000 article, “Root Water Uptake and Transport: Using Physiological Processes in Global Predictions,” in Trends in Plant Science (vol. 5). (back to top)
11 See note 4. (back to top)
12 See note 6. (back to top)
13 See T.C. Hsiao and K.J. Bradford’s 1983 article, “Physiological Consequences of Cellular Water Deficits,” in Limitations to Efficient Water Used in Crop Production (American Society of Agronomy, 1983). (back to top)
14 See R. Mittler et al’s 2001 article, “Living Under a ‘Dormant’ Canopy: A Molecular Acclimation Mechanism of the Desert Plant Retama raetam,” in The Plant Journal (vol. 25). (back to top)
15 See C.L. Guy’s 1990 article, “Cold acclimation and freezing stress tolerance: Role of protein metabolism,” in the Annual Review of Plant Physiology and Plant Molecular Biology (vol. 41). (back to top)
16 See A. Bertrand and Y. Castonguay’s 2003 article, “Plant Adaptations to Overwintering Stresses and Implications of Climate Change,” in Canadian Journal of Botany (vol 81). (back to top)
17 Ibid. (back to top)
18 See J.J. Volence et al’s 1996 article, “A Role of Nitrogen Reserves in Forage Regrowth and Stress Tolerance,” in Plant Physiology. (back to top)
19 See A. Bertrand et al’s 2001 article, “Molecular and Biochemical Responses of Perennial Forage Crops to Oxygen Deprivation at Low Temperatures,” in Plant, Cell, and Environment (vol. 24). (back to top)
20 Ibid. See also M.C. Drew’s 1997 article, “Oxygen Deficiency and Root Metabolism,” in the Annual Review of Plant Physiology and Plant Molecular Biology (vol. 48). (back to top)
21 For more, see D.A. Gaudet et al’s 1999 article, “Low-temperature Wheat Fungal Interactions: A Carbohydrate Connection,” in Plant Physiology (vol 106). (back to top)
Shazia Husain, PhD, is the research and development scientist at Vitaroofs International Inc. She has a doctorate from the Australian National University (ANU) and Commonwealth Scientific Industrial Research Organization (CSIRO) in the field of environmental sciences and molecular (stress) plant physiology. Husain has eight years of post-doctoral research experience at the Pennsylvania State University and the University of Toronto. Her recent work involves finding ways to improve the quality of growing media used for green roofs, checking the germination rate of the species used on the vegetation mats for their growth and survivability. Husain can be contacted via e-mail at shazia@vitaroofs.com.
To read about the Vancouver Olympic Village Green Roof, click here.