Changing the Language of Concrete: Communicating clearly with the project team
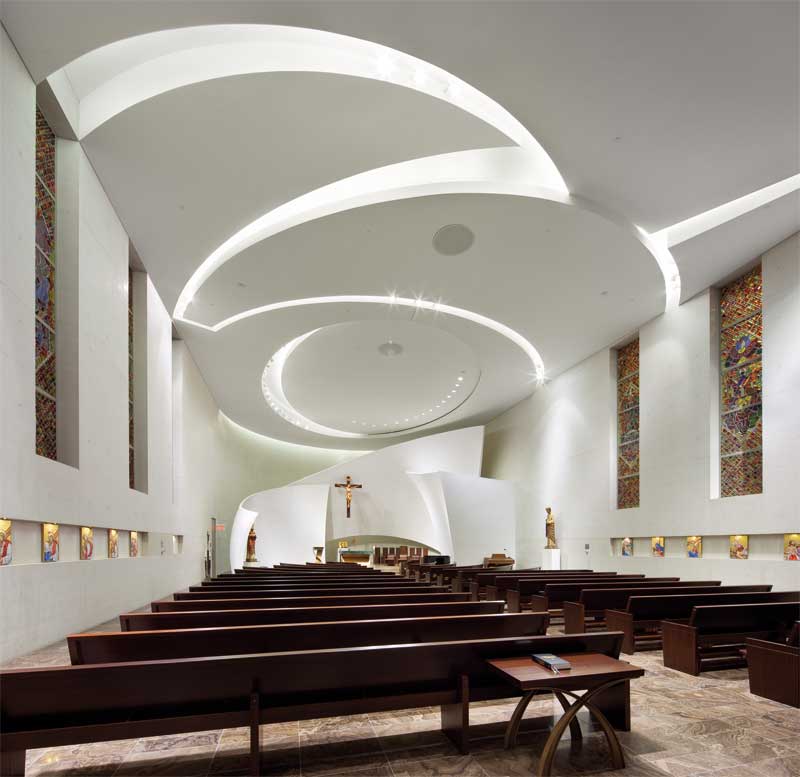
By Christopher Bennett, CSI, Assoc. AIA, Keith Robinson, FCSC, FCSI, RSW, LEED AP, and Rae Taylor, PhD
The Inuit have many different words for snow. ‘Aqilokoa’ means ‘softly falling snow,’ while ‘piegnartog’ describes ‘snow best for driving a sled across,’ and ‘pukak’ refers to ‘fine, powder-like snow on the ground.’ There are dozens more words describing frozen water in its many different forms. To those in warmer climes, having so many words for snow may seem superfluous, but to the Inuit, being able to both accurately communicate and describe their surroundings is a matter of survival and illustrative to their way of life.
In the construction world, accurately articulating concrete’s structure, form, and esthetics is crucial to success, yet not enough members of the project team are fluent in the material’s language. It is not uncommon to hear people use the terms ‘cement’ and ‘concrete’ interchangeably despite the obvious fact the former is a component of the latter. People with many years of construction experience often do not know different types of concrete exist and with very specific purposes (Figure 1).
Participants communicate differently
Design professionals and contractors speak differently about concrete, and it could even be argued they do not view the material’s end function the same way. Their approaches to communication vary because of how they approach their respective roles in construction, and are offset sufficiently that the path to clear instruction is lost.
Contractors approach communications pragmatically and require explicit instructions with expectations stated clearly and in detail, leaving no avenue for interpretation. Even when written specifications or drawings do not meet this expectation, their default position is to view the documents as a clear intention of fact.
Design professionals, on the other hand, deal with communications defined by implicit outcomes—with documentation prepared in a way that can be understood through often-unexpressed concepts. They rely on specifications and drawings that depend on references to standards, guidelines, and other sources not directly written. In other words, the instructions are not explicit and require the contractor to interpret potential outcomes based on the total content.
When it comes to concrete, its placement, and formwork, the design professional knows exactly what he or she wants, but may not fully appreciate the terms the contractor needs to price, purchase, and place the material. The design professional will name a project where concrete with an acceptable architectural finish can be found without understanding the conditions in which the representative sample was placed and how they differ from the current circumstances.
The contractor is looking for temperature, humidity, and other environmental conditions needed to make concrete look the way the design professional requires. Architects or engineers understand formwork and concrete mix influence esthetic outcomes, but they lack the practical experience to describe other controlling factors affecting the final placed concrete.
Even among design professionals, engineers and architects use different language, or—worse yet—try to employ each other’s terms to describe what they require. As one example, architects will use floor flatness (FF) and levelness (FL) to describe appearance outcomes for concrete slabs. The problems with this approach are further compounded when the architect uses the same descriptions for elevated slabs, where flatness values are greatly diminished and levelness does not apply.
What the architect is describing are requirements for floor smoothness and evenness—reducing the ripples and ridges associated with concrete floating and trowelling, as well as improving the planarity of the surface to reduce the degree of slope of the raised slab between structural bays. The engineer, however, uses the FF and FL values to describe expectations to control surface features that can potentially affect the safety of people using the floor surface, with the understanding there is limited applicability to floors not on-grade. Structural requirements do not typically align with architectural expectations, so this failure to communicate can cause problems unless discussions happen well in advance. With advance discussions, however, it is entirely possible architectural requirements can be clearly identified on structural drawings and in the specification content.
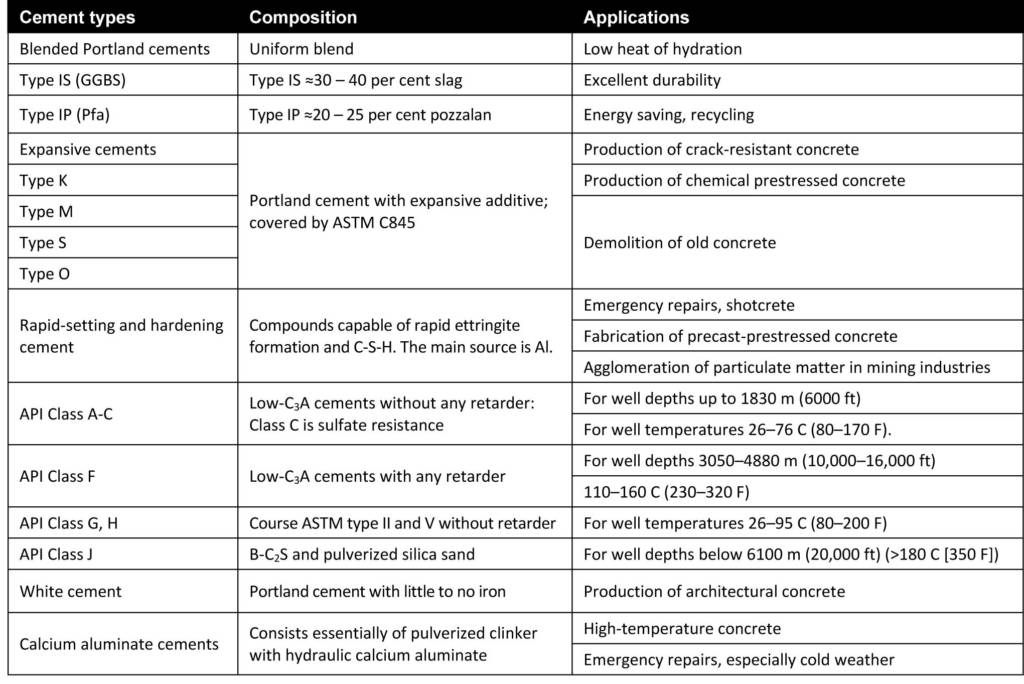