Assessing thermal performance and resiliency of contemporary buildings
by John F. Straube, PhD, P.Eng., and Brian J. Hall
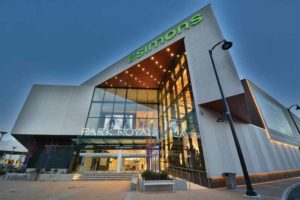
Requirements for energy and thermal performance and resilience are growing more stringent, as well as playing an increasingly significant role in building codes throughout North America. However, understanding and meeting the requirements has also become more complex for building designers. At the same time, it has become clear important decisions regarding basic structure, enclosure assembly, and window area need to be made early in the design process to achieve the most cost-effective, energy-efficient, and comfortable building. Designers, developers, and owners must be compliant with modern building codes, and familiar with suitable methods for quickly estimating, at an early stage, the thermal performance and resiliency of building systems.
Energy code
The 2017 edition of the National Energy Code of Canada for Buildings (NECB) is an important step toward Canada’s goal of achieving net-zero energy ready (NZER) buildings by 2030 for new construction, as presented in the Pan-Canadian Framework. NECB 2017 supports this goal by:
- reducing the overall thermal transmittance of roofs, fenestration, and doors;
- minimizing losses through thermal bridging in building assemblies; and
- limiting the allowable percentage of skylight area.
The new edition also introduced more stringent requirements for energy recovery systems and incorporated interior and exterior lighting requirements.
The current environment
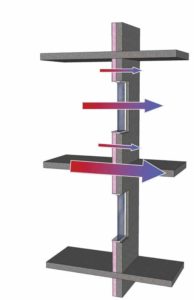
Images courtesy The Canadian Pre-cast/Prestressed Concrete Institute
Current Canadian and U.S. building codes are heavily influenced by energy considerations. This was not always the case. The American Society of Heating, Refrigerating and Air-Conditioning Engineers (ASHRAE) published one of the first building energy standards (ASHRAE 90.1, Energy Standard for Buildings Except Low-Rise Residential Buildings) in 1975. The earliest national standard for building energy performance, NECB, was first introduced in 1971 while the International Energy Conservation Code (IECC) was not launched in the United States until 2000.
Early on, neither ASHRAE 90.1 nor the other two model energy codes were widely adopted. In Canada, some provincial and municipal governments used NECB as the basis for design and construction of new public buildings. Institutions such as universities or large public companies also made compliance with NECB or ASHRAE 90.1 a requirement for the design and construction of an increasing number of high-profile buildings. As public awareness and concern grew over global warming, greenhouse gas (GHG) emissions, and other environmental issues, so did the prevalence of energy and environmental rating systems such as Leadership in Energy and Environmental Design (LEED). In time, rating programs, energy regulations, and model energy codes encouraged the evolution of building standards. Today’s codes integrate many of the energy and thermal performance requirements from earlier regulations and model codes. Dependence on traditional materials and enclosure systems has also changed.
The industry has adopted and continues to develop new and improved ways of building to respond to these changing code requirements and increasing performance expectations. Many different types of building systems are now being used throughout North America, and this has prompted the development of more accurate methods for the comparison and assessment of their actual in-service thermal performance. The focus on better methods of predicting heat flow has, or soon will have, entered mainstream building codes across North America. These methods of accounting for heat flow will impact all building enclosure systems.
The purpose of the “Meeting and Exceeding Building Code Thermal Performance Requirements for Precast Concrete Walls” guide by RDH Building Science is to allow design and energy modelling to proceed by estimating what thickness of insulation or changes in construction details would be required for specific R-value targets. The information is also intended to assist designers and owners in making better comparisons between systems in a project’s early stages (when many irrevocable decisions are made). Due to the specifics of the overall building design, the results may not be sufficient to demonstrate code compliance—additional energy modelling or trade-off analysis may be required. The guide summarizes various compliance paths for meeting building energy codes. As these paths include trade-off options, various levels of insulation can be used in walls for different projects. To accommodate this reality and simplify the document, thermal performance is provided for a range of insulation options. The thermal performance of select details repeated throughout a building is also considered.
The approach taken is to:
- begin with an overview of the current thermal performance requirements in the Canadian codes;
- provide an explanation of approximate methods to predict the thermal performance of common precast concrete systems for use during early design stages along with examples; and
- present examples of precast enclosure system solutions, and the calculation of their R-value, to meet the thermal performance requirements.