The next generation of passive solar for cold climates
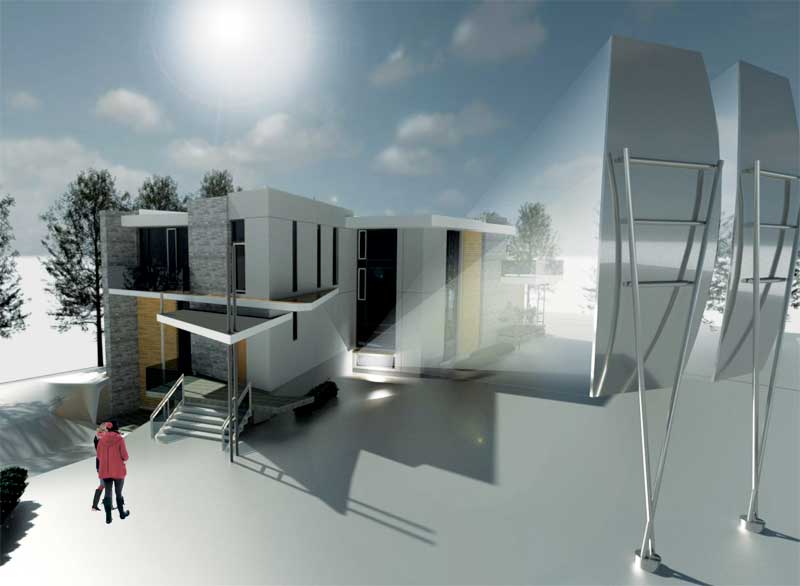
By Mark Driedger
As a child in the early 1980s, this article’s author had a clubhouse he called the ‘Millennium Falcon’—standing 2 m (6 ft) off the ground, it was a simple A-frame held in place by four old telephone posts, complete with a fireman’s pole and pully system for escape when under attack by the Imperial Starfleet. However, it was also an experiment in solar energy storage.
In its former location, the ‘Falcon’ had been filled with stones from the surrounding fields, and its south façade was fully glazed, allowing the sun to access the stones. The theory was the sun’s heat could be harvested from the stones long after it had dropped below the horizon.
The author’s parents’ plan was to build a house that was extremely energy efficient, and this was the prototype to provide the heating energy. In the early ’80s, natural gas pipelines had yet to reach the farm, and heating with electricity or fuel oil were the only options. With rising energy costs, it became important to save money, and using glazing to access passive solar energy was heavily featured in the media.
With the input of an American architect who had experience with passive solar design, traditional south-facing windows were chosen for the house, and a well-sealed staggered-stud enclosure was employed rather than the more exotic thermal mass method. Unfortunately, the chosen system simply was not balanced to provide additional energy to the house—although some sunlight was harnessed, the reality was the low thermal performance of this particular glazing caused much more energy to be lost than gained.
The glazing was also not large enough to collect sufficient heat, but increasing its size would also increase conduction losses during the cold winter nights, as glass is inherently a terrible insulator. Increasing the thermal properties of the windows reduced these losses, but it also reduced the sunlight that was allowed through. The system may have worked in a more temperate climate, but in Kingsville, Ont., the structure was destined to become the Millennium Falcon.
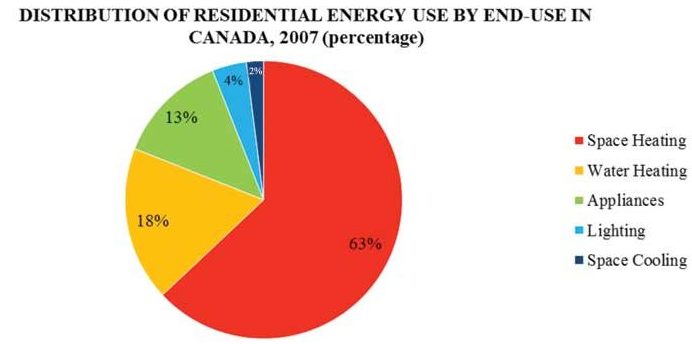
Some 30 years later, as an architect, this author’s interest in the problem remains. Why are architects not doing more to harness the sun? Yes, photovoltaics (PVs) have become more efficient, and large-scale battery installations are being considered, but battery storage still has a long way to go. Given a large portion of the energy used in buildings by Canadians is for heating (Figure 1), it would be very difficult and expensive to build a battery-storage system that would power a house through the winter. (This data was obtained from National Resources Canada (NRCan). For more information, click here.) It does not help that the cost of natural gas is still affordable at the moment, and it is cheaper for Canadians to run pipelines from Alberta than convert furnaces to electric heat and build solar panels with huge battery banks. Technology has changed rapidly over the last 30 years, but the equation still comes down to how energy from the sun can be efficiently stored. Research seems to be focused on the complex transformation of solar energy using batteries, rather than the simple transfer of energy into a thermal mass.