Galvanized reinforcing steel in precast concrete
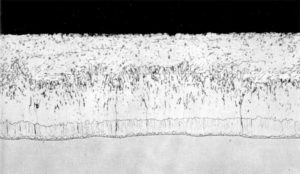
Traditional
The traditional method of galvanizing is performed to ASTM A767, Standard Specification for Zinc-Coated (Galvanized) Steel Bars for Concrete Reinforcement, Class I or Class II. The resultant coating is often comprised of alloy layers only and is at least 135 μm (5 mils) and 85 µm (4 mils), respectively.
Modified traditional
The modified traditional method is also performed to ASTM A767 Class II, but the coating is a minimum 85 μm thick and is made up of approximately 50 μm (2 mils) of pure zinc eta (the outermost layer) (Figure 2).
Continuous
The third is a continuous method produced to ASTM A1094, Standard Specification for Continuous Hot-Dip Galvanized Steel Bars for Concrete Reinforcement, and applies to nearly 100 per cent eta layer (i.e. pure zinc to a minimum coating thickness of 50 μm).
The differences in the coatings produced has implications for fabrication and service life, and will be discussed later in this article.
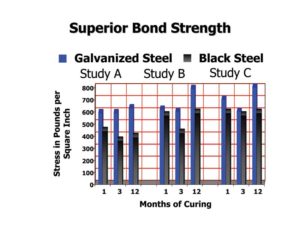
Galvanized coating structure
During the traditional galvanizing process, a series of alloy layers form as a result of the metallurgical (diffusion) reaction between the molten zinc and the steel. Figure 3 shows the cross-section of a coating developed on steel with a low silicon (less than 0.03 per cent). The coating consists of a thin gamma layer next to the steel substrate, a blocky delta layer, and a columnar growth of zeta crystals. The various alloy coatings contain different amounts of iron, with the highest content in the layers closest to the steel. The iron-zinc intermetallic layers are covered by a surface of pure zinc eta layer that is formed when the product is withdrawn from the molten zinc bath. This outer layer gives the galvanized product its distinctive shine and spangled appearance.
Measuring galvanized coating performance (corrosion rate) in concrete
By the nature of reinforcing steel being imbedded in concrete, it is difficult to assess the actual performance of the galvanized coating. The bar cannot be visually inspected without drilling core samples, and it is not as simple as measuring the electrical potential (measurements indicating the cathodic protection taking place [i.e. the zinc is giving up electrons to protect the steel]) to see how much corrosion is occurring. Zinc in concrete has different corrosion mechanisms than bare reinforcing steel. Regardless of how much engineers and scientists want to measure corrosion rates of galvanized rebar by accelerating one variable like chloride, doing so in accelerated tests such as salt fog or salt spray do not reflect what is taking place in a precast panel or barrier in the real world where it may take several decades before the chloride levels in the concrete are high enough to begin the zinc corrosion process. Parameters can be accelerated but the actual lifetime data in the test environment cannot be correlated to the field performance. Accelerated tests of chloride threshold for reinforcing bars have found zinc coatings have higher thresholds than bare steel by a factor of between five and 10 to one. This higher threshold, defined as the time when active corrosion of the zinc coating begins, is in part due to the formation of calcium hydroxyzincate film on the surface of the zinc coating during the concrete curing process, and indicates galvanized reinforcing steel should have a longer lifetime than bare steel. In addition to the higher threshold for chloride attack, the zinc corrosion products migrate away from the bar and fill the small capillaries and voids, thus preventing chloride ions from penetrating the concrete and coming into contact with the reinforcing steel. Black/bare rebar corrosion products such as iron-oxide are more voluminous than steel and cause spalling pressure, which eventually causes cracks at the concrete surface.
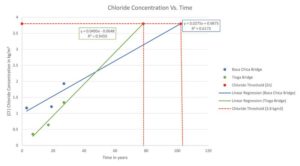
Based on coring of two bridge decks in different exposures, as indicated in Figure 1 (page 29), the chloride threshold for the galvanized rebar is not reached until year 78 and 102 for a bridge in a northern climate (Tioga bridge, Pennsylvania) where road salts are used and for a bridge in a southern climate (Boca Chica bridge, Florida) with marine exposure, respectively. Conversely, the chloride threshold for black/bare bar would be reached sometime around 10 to 15 years after the concrete pour (Figure 4).
It is important to note after the chloride threshold is reached, the galvanized coating still protects the substrate bar for additional years, as a function of the zinc coating thickness. Depending on whether bar was produced by the traditional method to ASTM A767 Type I, the modified traditional method to ASTM A767 Type II, or via the continuous method to ASTM A1094, protection may be extended for several decades beyond the chloride threshold point (Figure 5). Longer protection means little or no maintenance costs for a bridge deck. The galvanized rebar will usually last much longer than the life of the concrete it is giving strength to.
What precasters should know
Significant tonnage of galvanized rebar is used by precasters to give their products a longer life, but certain performance characteristics of galvanized rebar should be known in advance to maximize
its value.
Forming and fabrication of galvanized rebar
Welding, cutting, and drilling of the steel should be done prior to galvanizing to minimize the exposure of unprotected edges and to take advantage of the protection afforded by the zinc coating. Good fabricating practices state, before galvanizing, steel should be bent with a minimum six to 10 times the bar diameter, per Table 2 of ASTM A767. Heat-treating before bending is required for bends tighter than that. This bend radius minimizes possible cold working of the steel structure that could lead to strain-age embrittlement after galvanizing. Strain-age embrittlement sometimes occurs when bending stresses are induced in the steel fabrication process and then released by the heat in the galvanizing process. The galvanized steel becomes frail.