Exploring John Abbott College’s latest building
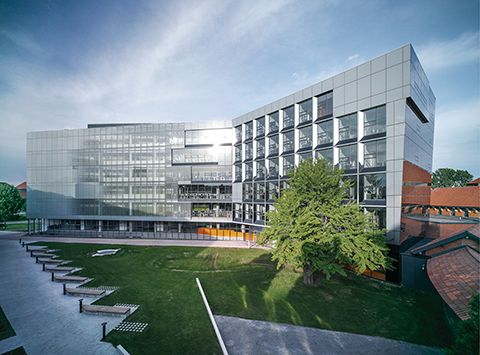
By Pierre-Luc Baril, Eng., HFDP, LEED AP, and Nicolas Lemire, Eng., M.A.Sc., LEED AP
A tree must first take its strength from its roots, and a successful building must do the same. It is not possible to throw in some fancy ‘green’ devices once everything else has been decided to render a building ‘sustainable’ or simply add up various eco-measures and get a high-performing, energy-efficient facility. For a true sustainable result, it must come from the early stages of a project, with the roots guiding every decision. This simple analogy explains how John Abbott College approaches its new science and technology building project. It is also fitting because tree roots came into play for the school’s new design.
In the early 2000s, the college—located in Sainte-Anne-de-Bellevue, near the western tip of the Island of Montréal—was struggling to keep an edge with its sciences program because of the lack of proper space to teach an increasing number of students. Laboratories and classrooms more than 40 years old were too small and inadequate for contemporary demands and ways of teaching.
At this point, John Abbott College was in serious need of additional specialized, square footage. The school required a new building to teach physics, biology, and chemistry, as well as professional programs for nursing, paramedic, and the newly approved biopharmaceutical studies program. Further, the lack of adequate swing space rendered the renovation of the existing teaching space to be quite difficult.
To put things into perspective, John Abbott College is located on a historic campus with buildings dating back to the early 1900s. To fit a new construction inside this setting without having it look out of place was a serious challenge. Also, it was really important to reduce the impact of this new building in terms of environmental footprint.
Early on, the school decided to hire a complete set of professionals to discuss the possibility for this project and ensure the building would make a clear statement to the community about how important the environment is for future generations. The selection process included a first submission by potential architectural and engineering firms followed by specific interviews conducted by a multidisciplinary committee. At the end of the process, Saucier + Perrotte architectes was teamed up with Pageau Morel et associés inc. (mechanical/electrical/plumbing [ME]) and SDK et associés inc. (structural/civil). SNC-Lavalin served as project manager.
The new building
An integrative design process was established with the stakeholders and the team. This translated in several ‘charettes’ to discuss the ideas, obstacles, and opportunities related to the project. Goals were defined in terms of performance and sustainability for the new building. The potential construction site was quickly identified, but was limited by the existing building, a semicircle passage joining several buildings, and a Ginkgo tree located in the middle of the courtyard.
The tree was more than a century old, and the team felt it would be a powerful statement to incorporate its presence in the design of the new building. In other words, there needed to be a way to integrate the building design with the existing environment, and not the other way around.
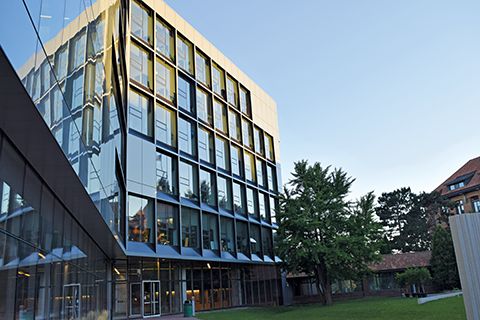
The new Anne-Marie Edward Science and Health Technologies Building is a six-storey facility with a gross area of 11,280 m2 (121,416 sf) and a net interior area of 6200 m2 (66,736 sf). It contains classrooms, faculty offices, teaching laboratories with chemical fumehoods, social spaces, and a large atrium connecting every floor. This building is shaped at a 40-degree angle to fold around the Ginkgo. Also, the main staircase of the building, centrally located and fully glazed to the exterior, is designed to emulate the tree, with its branches extending upward. Fully glazed curtain wall and large windows were installed on a significant portion of the building to maximize natural light and views so the occupants could easily connect with the exterior.
This building was named after Anne-Marie Edward, a John Abbott graduate and engineering student who was one of the victims of the 1989 shooting at École Polytechnique in Montréal. The school felt the new science building, through engineering and applied sciences, showed how humans must contribute to environmental sustainability by using applied knowledge and technology.
Technical features
Since this building had a lot of energy-intensive laboratories, it was paramount to include, in the early stages of design, advanced computerized energy calculation to evaluate different possibilities for overall energy consumption. The best-performing option considering the use of the building within the available budget included geothermal wells, thermal storage, radiant heating and cooling, and a decentralized fan coil network combined with efficient ventilation units.
The simulation based on the 1997 Canadian Model National Energy Code for Buildings (MNECB) predicted the building would use 39 per cent less energy than the baseline. Once the building was completed, real energy usage was monitored for the first year of operation and the results exceeded expectations by 10 per cent. In fact, actual energy use in the building is 45 per cent lower than the baseline case. Annual site energy intensity is currently 151 kWh/m2, whereas the baseline is 275 kWh/m2.
Key mechanical elements helped contribute to the building’s energy efficiency. For example, a hydronic network creates and distributes the energy all around the building; it is truly of vital importance to the building operation. This network is composed of 40 geothermal wells, each around 120 m (394 ft) deep. They are used to reactivate two 3000-L (792.5-gal) thermal storage tanks. These tanks—one cold and one hot—are connected to five two-stage heat pumps for a total capacity of more than 175 tonnes. They can simultaneously produce hot and cold water for the building needs.