Designing thermally comfortable buildings
Madeleine Lunde, EIT, and Marc Trudeau, P.Eng., Architect AIBC, BEMP, CPHD, LEED AP
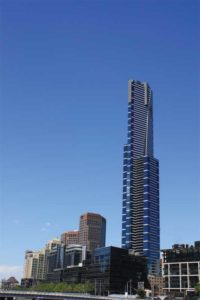
Buildings are designed for the people who live and work within them. Yet, more than 40 per cent of occupants of office facilities have reported dissatisfaction with their thermal environment (For more information, read Percentage of commercial buildings showing at least 80% occupant satisfied with their thermal comfort by Caroline Karmann, Stefano Schiavon, and Edward Arens (2018), proceedings of 10th Windsor Conference: Rethinking Comfort). This is because building design often disregards thermal comfort due to the analysis involved in quantifying a person’s subjective human response to the surrounding conditions. Thermal comfort is defined as “the condition of mind that expresses satisfaction with the thermal environment,” and is, therefore, experienced differently by each person (This definition is from the American National Standards Institute, American Society of Heating, Refrigerating and Air-conditioning Engineers (ANSI/ASHRAE) 55-2017, Thermal environmental conditions for human occupancy). Thermal comfort is a personal interaction between how one’s body generates metabolic heat (thermoregulatory response and activity level) and how that heat can be lost because of clothing insulation and environmental conditions. Ensuring people can achieve thermal equilibrium within a space is essential to their satisfaction, workforce productivity, and overall health and well-being.
When a person feels uncomfortable with the indoor environment, they will seek to alleviate that discomfort through measures such as opening a window when hot or using a personal space heater when cold. This can result in suboptimal building operation that could cause excessive energy use and additional operational costs. If thermal comfort is not considered in the design process, the building is more likely to be improperly operated because of unsatisfied occupants responding to their discomfort. If thermal comfort issues can be identified prior to occupancy, it is possible to optimize building design such that passive and energy-efficient strategies can be incorporated rather than relying solely on HVAC systems to meet (or not meet) personalized thermal comfort demands.
While the goal is to achieve 100 per cent thermal satisfaction for every building space, this is an impossible goal due to the large variations, physiologically and psychologically, from person to person (This definition is from the American National Standards Institute, American Society of Heating, Refrigerating and Air-conditioning Engineers (ANSI/ASHRAE) 55-2017, Thermal environmental conditions for human occupancy). However, with careful analysis and design, comfort can be achieved for the vast majority of occupants without having to alter the operations of the space.
ASHRAE 55
Due to the complexity of designing for thermal comfort, guidelines have been developed to help building professionals achieve thermal comfort within occupied building spaces. The American Society of Heating, Refrigerating and Air-conditioning Engineers (ASHRAE) 55, Thermal Environmental Conditions for Human Occupancy, is the industry standard by which North American building codes, incentive programs, and certifications base their requirements. ASHRAE 55 provides analytical methods of evaluating the interaction between the following six conditions relating to thermal comfort:
- metabolic rate (met);
- clothing insulation (clo);
- air temperature;
- mean radiant temperature;
- average air speed; and
- relative humidity (RH).
These factors, when considered concurrently and in steady state, can depict an accurate forecast of the thermal satisfaction of future building occupants. ASHRAE 55-2017 predicts thermal comfort through the adaptive thermal comfort (ATC) and whole-body thermal-balance comfort (WBC) models.
Adaptive thermal comfort model
ATC is used in naturally ventilated buildings and suggests a range of acceptable indoor operative temperatures based on mean outdoor temperatures with the assumption occupants can adapt to their environments as necessary. To comply with this method, an 80 per cent acceptability limit must be achieved (i.e. 80 per cent of occupants in a naturally ventilated space must be satisfied with the thermal conditions). This method can be used when the mean outdoor temperature is greater than 10 C (50 F) and less than 33.5 C (92 F). The allowable indoor operative temperature can be determined using the 80 per cent acceptability limits from Figure 1, which is taken from Figure 5.4.2 of ASHRAE 55-2017 (This definition is from the American National Standards Institute, American Society of Heating, Refrigerating and Air-conditioning Engineers (ANSI/ASHRAE) 55-2017, Thermal environmental conditions for human occupancy).
The focus of this model is to ensure naturally ventilated buildings, typically with improved building envelopes, do not overheat due to lack of design strategies that limit heat gain, and promote passive cooling. This method encourages the implementation of passive design strategies. Some of the strategies are operable windows, exterior shades, interior blinds, and windows with low solar heat gain co-efficient (SHGC) and low shading co-efficient (SC) to reduce the risk of overheating in summer months.
Whole-body thermal comfort model
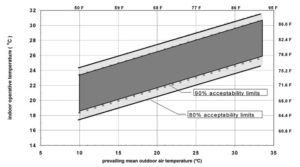
For buildings where mechanical conditioning is used, the WBC model is required, as it is assumed people will rely on building conditioning systems rather than natural ventilation for thermal comfort. Unlike the adaptive thermal model, the WBC one considers personal factors explicitly, requiring that building occupants have metabolic rates typical of indoor office activities (1.0 to 2.0 met) and clothing with thermal insulation of 1.5 clo or less (This definition is from the American National Standards Institute, American Society of Heating, Refrigerating and Air-conditioning Engineers (ANSI/ASHRAE) 55-2017, Thermal environmental conditions for human occupancy). The WBC model aims to predict the thermal sensation and dissatisfaction of occupants in a space employing a predicted mean vote (PMV) and predicted percentage dissatisfied (PPD). The PMV model uses heat balance principles to relate the six governing conditions of thermal comfort to the average response of occupants based on a thermal sensation scale, defined as follows: +3 hot, +2 warm, +1 slightly warm, 0 neutral, – slightly cool, –2 cool, and –3 cold. People voting hot, warm, cool, or cold are considered thermally dissatisfied. PPD is an important index. It is calculated using PMV, and is employed to determine if a building will meet acceptability limits. The thermal comfort range is defined as –0.5 < PMV < 0.5 and PPD ≤ 10 per cent, which corresponds to an 80 per cent acceptability limit (This definition is from the American National Standards Institute, American Society of Heating, Refrigerating and Air-conditioning Engineers (ANSI/ASHRAE) 55-2017, Thermal environmental conditions for human occupancy).
Codes and certifications
Thermal comfort is referenced in global building rating systems, such as the Leadership in Energy and Environmental Design (LEED), WELL, and Passive House, as well as Canadian building codes such as the B.C. Energy Step Code and the City of Vancouver Energy Modelling guidelines. To meet these codes and certifications, it is required to show plots and calculations verifying the design parameters meet the specified thermal comfort requirements. Thermal comfort is not currently a requirement of the National Energy Code for Buildings (NECB) and is therefore only considered in buildings pursuing relevant green building certification credits (e.g. LEED) or in provinces that have elected to enact regulations relating to thermal comfort.
LEED v4 provides one credit in Indoor Environmental Quality for thermal comfort. This credit requires the design of HVAC systems and building envelopes to meet the requirements of either ASHRAE 55-2010 or the International Organization for Standardization (ISO) and the European Committee for Standardization (CEN) standards. For building types, such as schools and health-care projects, group thermal comfort controls must be provided for all shared multi-occupant spaces and individual comfort controls must be provided for at least 50 per cent of individual occupant areas. Thermal comfort controls should allow occupants to adjust at least one of the following in their local environment: air temperature, radiant temperature, air speed, and humidity.
WELL v2 puts an even greater emphasis on thermal comfort compared to LEED, with one prerequisite, six credits, and two beta credits used to evaluate thermal comfort. The thermal performance prerequisite requires compliance with the 80 per cent acceptability limit for naturally ventilated spaces and –0.5 < PMV < 0.5 and PPD ≤ 10 per cent for 95 per cent of regularly occupied mechanically ventilated spaces, as per ASHRAE 55-2013. WELL provides thermal comfort points if any of the following additional credits can be achieved:
- enhanced thermal performance: 90 per cent acceptability limit and successful post-occupant surveys;
- thermal zoning: thermostat controls present in every thermal zone and free address for employees;
- individual thermal comfort: access to personal thermal comfort devices and facilitation of thermal adaption such as flexible dress codes;
- radiant thermal comfort: implement radiant systems and dedicated outdoor air systems;
- thermal comfort modelling: monitor dry-bulb temperature, RH, air speed, and mean radiant temperatures in occupied spaces; and
- humidity control: maintaining relative humidity levels between 30 and 60 per cent.
WELL continues to make advancements on what it means to achieve thermal comfort. Two credits that encourage enhanced operable windows and outdoor thermal comfort are currently in beta testing. WELL has a stringent and in-depth thermal comfort criterion, which makes it an important resource to reference when prioritizing thermal comfort design, regardless of the pursuit of a WELL certification.
Project analysis
As an example, thermal comfort targets were required on a multilevel student residence building. This project illustrates the analysis and strategies that are involved in designing for thermal comfort.
Targets
The project had multiple comfort targets.