Balancing building science and roof design
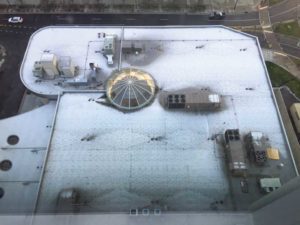
By James R. Kirby, AIA
The design and construction industries are increasingly using building science concepts to design and construct long-lasting, high-performance buildings. The concepts are not new and have been understood by some in the building and design communities for decades. However, for many designers, it is a shift from traditional practice emphasizing form, esthetics, and programmatic requirements over building science fundamentals.
Understanding the complex nature of building enclosure performance and the science behind it will have a positive impact on how buildings and roofs are designed, constructed, and endure. Building science also assists architects in mitigating the risks of design-related building failures.
Understanding building science
Building science is the study of the effects of heat, air, and moisture (vapour and liquid) on the building enclosure. It involves an understanding of building performance within specific climate and environmental conditions (Figure 1) from a systems perspective rather than as a collection of individual unrelated components.
Building science can be used to mitigate or prevent damage from air and moisture infiltration and high winds, and to improve the energy efficiency, durability, and long-term performance of buildings.
Second law of thermodynamics
The fundamentals of building science are found in the Second Law of Thermodynamics:
∞ Heat flow is from warm to cold;
∞ Moisture flow is from warm to cold;
∞ Moisture flow is from wet to dry;
∞ Air flows from higher pressures to lower pressures; and
∞ Gravity acts down (think Isaac Newton’s apple tree).
In short, energy, air, and moisture are exchanged between two systems until a state of equilibrium or balance is achieved (Figure 2).
The struggle with water
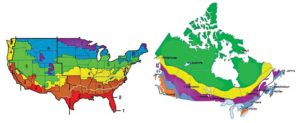
Of the many challenges a building enclosure faces, water represents the greatest continuous threat. The presence of water in unwanted locations in the building enclosure can contribute to rot, decay, and bio growth, as well as a reduction in thermal efficiency. In other words, while high-wind events, impacts, and fires must be considered in design, ongoing water infiltration should be of primary concern to building designers.
There are four primary moisture transport mechanisms to consider when designing a building’s enclosure: bulk water, capillary water, air-transported water, and vapour. The most obvious and impactful is bulk water, such as rain. Buildings must be designed to efficiently shed or manage liquid water, and the design community generally does a good job of properly detailing the building enclosure to achieve this.
Next is capillary action, which primarily deals with below grade moisture infiltration through pores and small cracks in foundations and the intersections of above- and below-grade systems. The design community does a good job of properly detailing the building enclosure for capillary action, as well.
The last two—air-transported water and vapour diffusion—influence the performance and durability of building enclosures, but the materials and designs used to control air intrusion and reduce vapour diffusion are often less obvious.
Air leakage can carry 10 to nearly 100 times the amount of moisture when compared with vapour diffusion, which is why the International Energy Conservation Code (IECC) has required the use of air barriers in building enclosures since 2012. Gaps and discontinuities in air barriers within the building enclosure (including the roof) allow air flow, with its associated moisture. This moisture intrusion can be problematic to say the least.
In contrast, vapour diffusion through building materials is less impactful than air intrusion and its carried moisture. For this reason, codes are nearly silent on the use of vapour retarders in roofs. However, the need for vapour retarders in certain climate zones and high humidity environments has been historically recognized by the design community, yet oftentimes these vapour retarders were beneficial because, unknowingly, they also acted as an air barrier. Due to a lack of building science knowledge, vapour retarders have not always been incorporated into designs correctly, causing building damage.
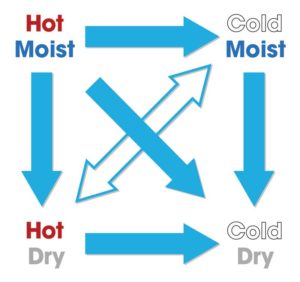
The effects of air movement and pressures within the building enclosure
Controlled and uncontrolled interior air pressures from wind, stack effect, and HVAC systems often combine to create air flow across the building enclosure (Figure 4).
First, wind exerts pressure on the building enclosure. On the windward side there is positive pressure, and on the leeward side there is negative pressure. The stack effect is about warm air rising, increasing pressures higher up in the building. There are also mechanical-related pressures caused by forced air systems.
These pressure differentials create air and moisture movement within a building and through, or across, its enclosure. Proper placement of the thermal layer and air/vapour control layers can help prevent moisture intrusion into the building enclosure. Building science can provide ways of predicting moisture movement within an enclosure and help determine which design configurations minimize or block vapour and moisture intrusion and retention.
The importance of managing uncontrolled air flow, and the moisture it carries, cannot be overstated. The air’s ability to hold moisture is dependent on its temperature. Warm air holds more moisture than cold air, and when warm moist air encounters a cold surface, the air will cool (and its ability to hold moisture will decrease). The excess moisture will condense on the cold surface.
Therefore, air barriers are best located on the warm side (in winter) of the dew point; proper placement of air barriers limits the risk of condensation on surfaces within the building enclosure. For roof systems, installing the vapour retarder as an air barrier (i.e. sealing all penetrations and transitions) allows one to address vapour diffusion and air transported moisture.