Improving the performance of curtain walls
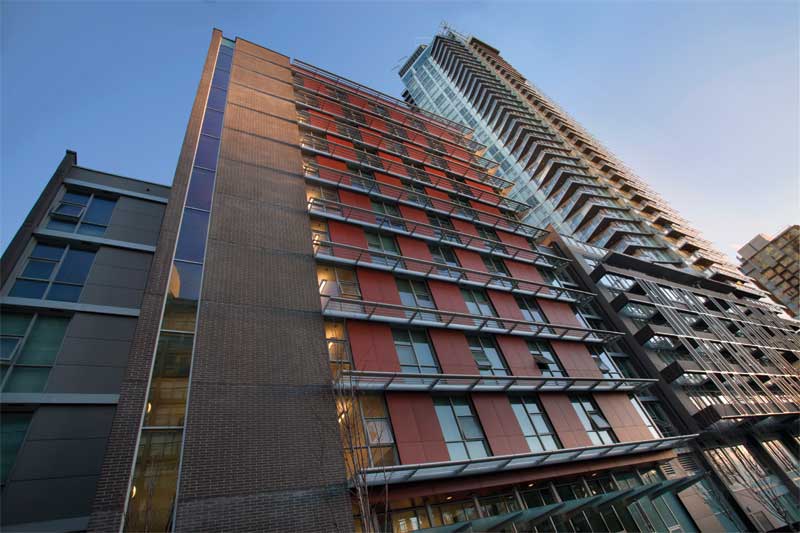
By Ben Mitchell, Chad Ricker, and Jerry Schwabauer
There is perhaps no building element that typifies contemporary architecture more than the curtain wall. Lightweight and flexible, these glazed assemblies not only allow more usable floor space within a building, but also epitomize upscale, modern construction. Widespread use of the curtain wall has driven various improvements in its functionality and sustainability, and recent improvements have focused mainly on thermal performance and coating technologies.
The North American Fenestration Standard (NAFS), a voluntary standard recently adopted into most Canadian building codes, has streamlined some aspects of the fenestration industry. However, the National Building Code of Canada (NBC) and some provincial codes distinguish between products covered by NAFS and those that are not—and the distinctions may not be intuitive.
For example, curtain wall assemblies are not manufacturer-tested or certified to be NAFS-compliant. Instead, engineers and architects are responsible for designing all individual structures to meet relevant energy efficiency goals. The portion of NBC applicable to curtain walls is Part 5, “Environmental Separation.”
Addressing issues such as air leakage and water penetration, Part 5 sets forth “minimum performance requirements, as well as laboratory and in-situ testing procedures,” according to the National Research Council of Canada (NRC).
Situation-specific testing and field assessments of curtain walls help ensure maximum energy performance in Canada’s cold northern climate. Designers must also provide protection against condensation, since condensation-related mould and moisture can create problems, particularly in sensitive environments such as healthcare facilities. In 2015, the American Architectural Manufacturers Association (AAMA) released AAMA CRS-15, “A Comparison of Condensation Rating Systems for Fenestration,” which compares three common condensation rating systems for the United States and Canada, providing information on how the ratings are calculated to help designers better assess the condensation resistance of fenestration systems.
Understanding the curtain wall
There are two main categories of curtain walls:
1. Stick-built systems, in which all structural components are assembled onsite. This system is optimal for building designs with little repetition among components, where no notable savings can be achieved by factory production.
2. Unitized curtain walls, in which glazed and aluminum components are factory-assembled into panels and shipped to the construction site. This method has several advantages, as the controlled factory setting improves material tolerances, allows faster onsite construction, and works well for tall structures with many repeat components.
The extruded aluminum members comprising the curtain wall frame can be two separate pieces (one for the building interior and one for the exterior) joined at pressure points, as is common in stick-built systems, or a single extruded profile, which is more common for unitized systems. While many curtain wall components are factory-assembled, overall NBC categorizes curtain walls as ‘site-built.’
Contributions to thermal performance
Throughout the 20th century, a series of improvements to glazed systems have reduced the rate of unwanted heat loss and gain for building interiors.
Window glazing
Single-pane window glass was commonly used well into the middle of the century. However, the proliferation of skyscrapers—and the extreme amount of thermal transfer occurring over their extensively glazed exteriors—prompted the commercial production of double- and triple-glazed insulating units in the 1940s and ’50s. Thermal isolator gaskets were also installed around metal parts such as mullions and pressure plates to insulate them and protect against air and moisture penetration.
In the 1980s, insulating glass (i.e. double panes with inert gas or a vacuum seal between them) enabled further reduction of heat transfer. Following that, low-emissivity (low-e) and other coatings for glass were developed. Together, low-e coatings and insulating glass (IG) units immensely improved the thermal performance of glazed openings, since the glass itself represents the largest surface area over which thermal transfer occurs.
Spacers
The most significant thermal path or ‘bridge’ remaining in glazed openings following these improvements was the spacer. In its earliest and simplest configuration, the IG unit consisted of two panes or ‘lites’ separated by an aluminum or metal spacer, which was held in place by seals. IG units used in curtain walls were dual-sealed, with polyisobutyl (PIB) primary sealants applied directly to the glazing and silicone used secondarily to provide a structural component. Spacers were usually U-shaped, with a desiccant placed within the canal to absorb any moisture between the lites.
This construction provided structural strength. However, it also provided a conduit of metal allowing heat and cold into the building, and created a temperature differential between the centre and the edge of the glass, which led to condensation. Replacing the traditional aluminum spacer with warm-edge spacers (i.e. those constructed from materials such as polymers or low-conductivity stainless steel) was a first step toward improving framing. Today, warm-edge spacers are typically an integral part of fenestration systems.
Thermal barriers
Even after the introduction of IG units and warm-edge spacers, the aluminum profile of the typical curtain wall assembly allowed significant remaining pathways for thermal transfer, since alloyed aluminum is highly conductive. However, aluminum is also prized for its many advantages—most notably, its durability, recyclability, and strength. It is a primary building material in sustainable buildings and those certified under the Leadership in Energy and Environmental Design (LEED) program. Therefore, the fenestration industry turned its attention to mitigating aluminum’s conductivity and, for both stick-built and unitized curtain walls, developed engineered systems capable of interrupting the thermal bridge created by the metal cross-sections of the assemblies.