Corporate Confidential: Understanding acoustic privacy within the built environment
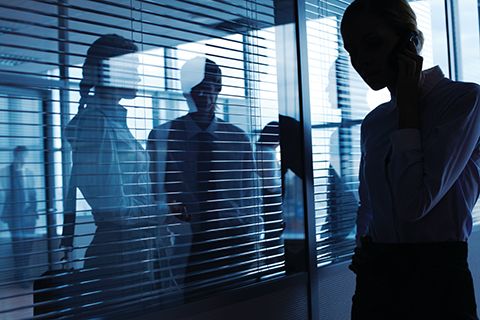
By Niklas Moeller
Typing the word ‘privacy’ into any search engine yields a virtually endless stream of entries describing the ways in which it can be violated. There are reports of hackers acquiring credit card information, law enforcement agencies mining social networking sites, and members of the public using drones to take aerial photographs. More recent headlines indicate voice-activated televisions can even eavesdrop on owners.
The preoccupation with vulnerabilities exposed by the Internet and electronic products is understandable given their relatively rapid spread into almost every aspect of everyday life. However, privacy can still be violated in ‘traditional’ ways. In fact, it can even be lost to those who do not intend to infringe upon it. People are often exposed to sensitive information simply by being within audible range of a conversation.
Current privacy legislation tends to focus on securing access to information stored on computers or within filing cabinets, but attention also needs to be paid to the built environment. When examined in this context, privacy has both an acoustic and a visual component. (This article primarily focuses on the former, except insofar as it is affected by the latter.)
What is acoustic privacy?
Many people immediately equate acoustic privacy with speech privacy, but there is more to this concept than the ability to clearly hear what another person is saying.
For example, if the conversation taking place in a room next to an occupant is unintelligible, one may still be able to identify the speaker’s tone and determine whether they are happy, sad, or angry. This type of information can be considered private under certain circumstances, such as when coming from behind the closed door of a human resources manager’s office—the same can be said for non-verbal noises like those overheard from an adjacent hotel room.
How much of a conversation is understood also depends on whether or not the speaker can be seen. This effect—known as visual cues—has been quantified by various studies.1 Generally speaking, if one can only understand 20 per cent of someone’s conversation when not looking at them, the ability to see their lips increases that amount to nearly 55 per cent. If you start at 50 per cent, visual cues increase it to almost 90. In other words, there is also a visual component to acoustic privacy, which is important to bear in mind when designing a space.
Further, acoustic privacy should not only be considered from the speaker’s perspective, but also that of the listeners. The reasons will become clear as this article explores the various impacts of a lack of privacy.
Where is it needed?
A lack of acoustic privacy carries real risk, particularly in facilities where there is a perceived need for it or an expectation on the part of its users. Examples include hospitals, bank branches, law offices, government, and military facilities. However, other types of spaces—such as commercial offices, call centres, and hotels—have privacy needs as well. The degree required typically depends on the type of activities the space hosts.
Why is it needed?
It is easy to understand the need for acoustic privacy—or even acoustic security—from a speaker’s perspective, particularly in environments where medical information, financial planning, personal relationships, trade secrets, or matters of national security are being discussed. However, a lack of acoustic privacy can have impacts beyond divulging sensitive information to unintended parties. This fact becomes clear when perspective shifts from the person talking to the involuntary listener.
When a noise or voice enters an occupant’s ‘space,’ some degree of annoyance is typical, but it can also make one feel as though one’s privacy—or sense of physical separation—is being invaded. Perhaps the most relatable examples of this sensation are when the guest in a neighbouring hotel room turns up the television’s volume or the patient at the other end of a waiting area starts speaking loudly into his or her cell phone.